Tăng cường giá trị dinh dưỡng của ngô bằng công nghệ sinh học
Nhu cầu về ngô ngày càng tăng trên phạm vi thế giới và Việt Nam. Ngô cũng giống như các cây ngũ cốc khác có hàm lượng một số chất dinh dưỡng quan trọng thấp, đặc biệt là các chất như lysine, vitamin A, acid folic, sắt, kẽm và selenium. Chính vì thế, các nhà khoa học đã có nhiều nỗ lực trong nghiên cứu nâng cao giá trị dinh dưỡng của cây ngũ cốc nói chung và cây ngô nói riêng. Bài báo này tổng quan các kết quả nghiên cứu về cải tiến dinh dưỡng của cây ngô bằng công nghệ sinh học. Trong đó tập trung vào các công trình cải tiến chất lượng protein, hàm lượng carotenoid và các vi dưỡng chất như sắt và kẽm. Những kết quả nghiên cứu này đã mở ra triển vọng cho việc cải tiến chất lượng dinh dưỡng ở cây ngũ cốc, trong đó có cây ngô nói riêng; đồng thời cũng cho thấy những vấn đề cần được tiếp tục nghiên cứu về hướng này.
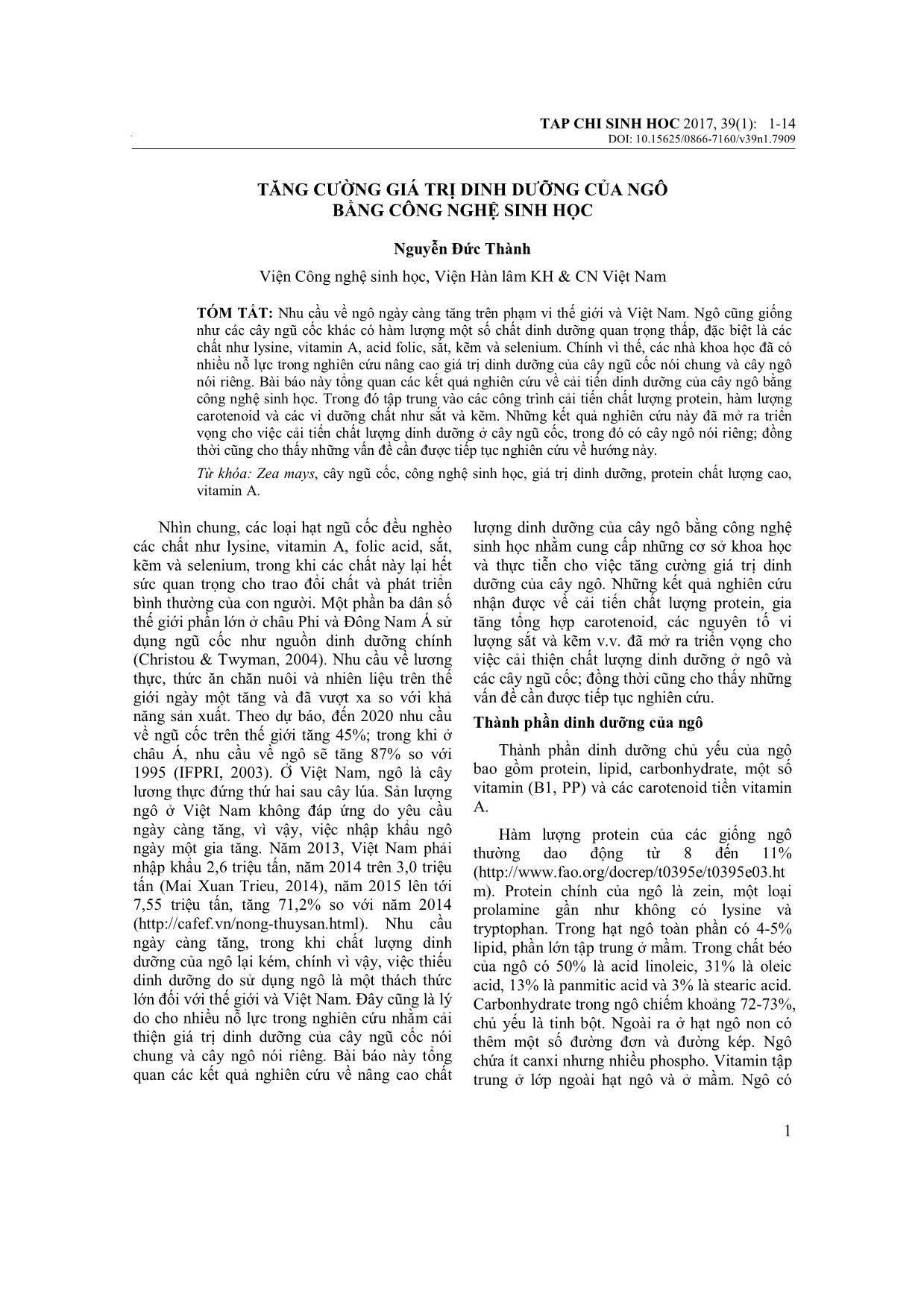
Trang 1
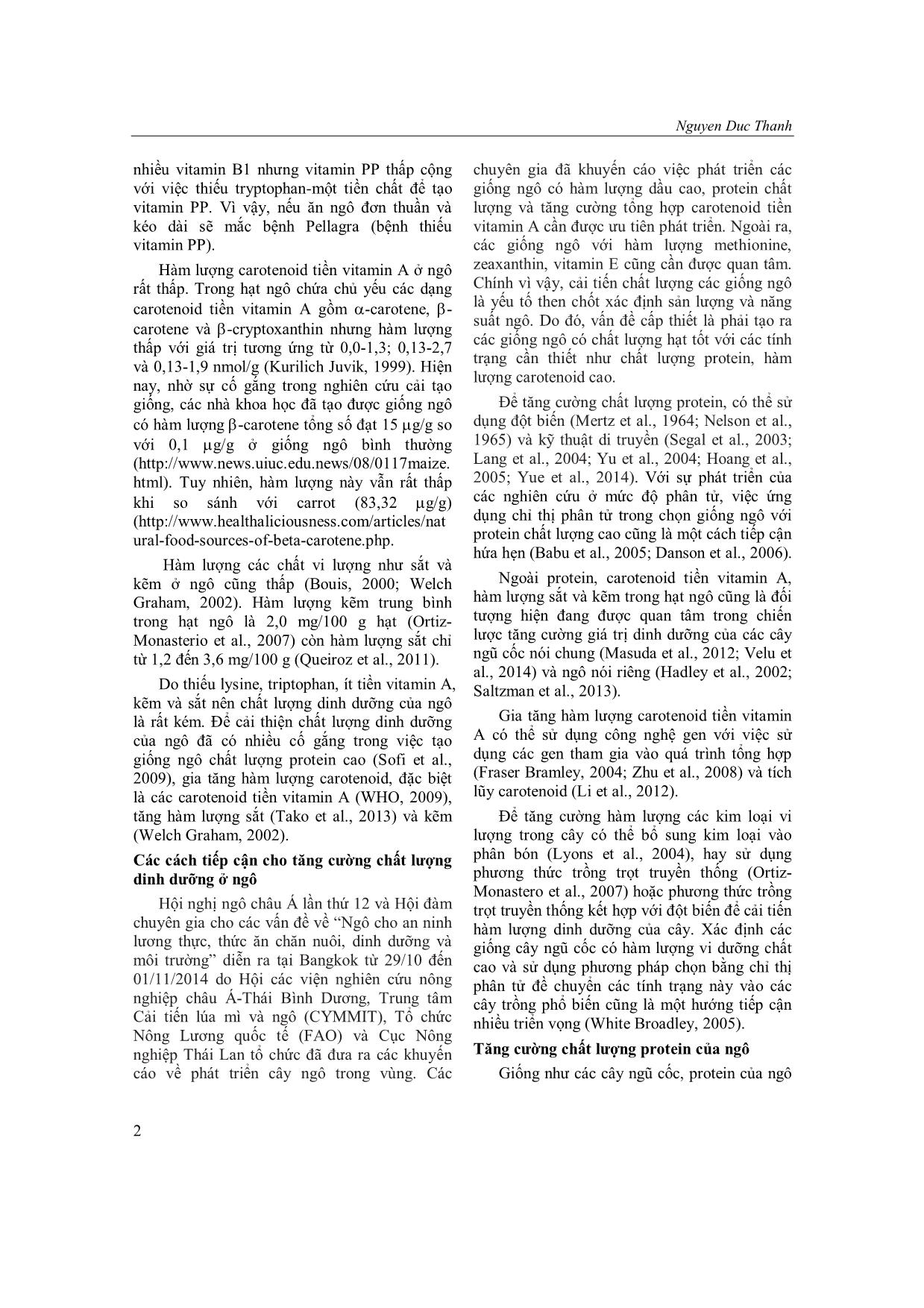
Trang 2
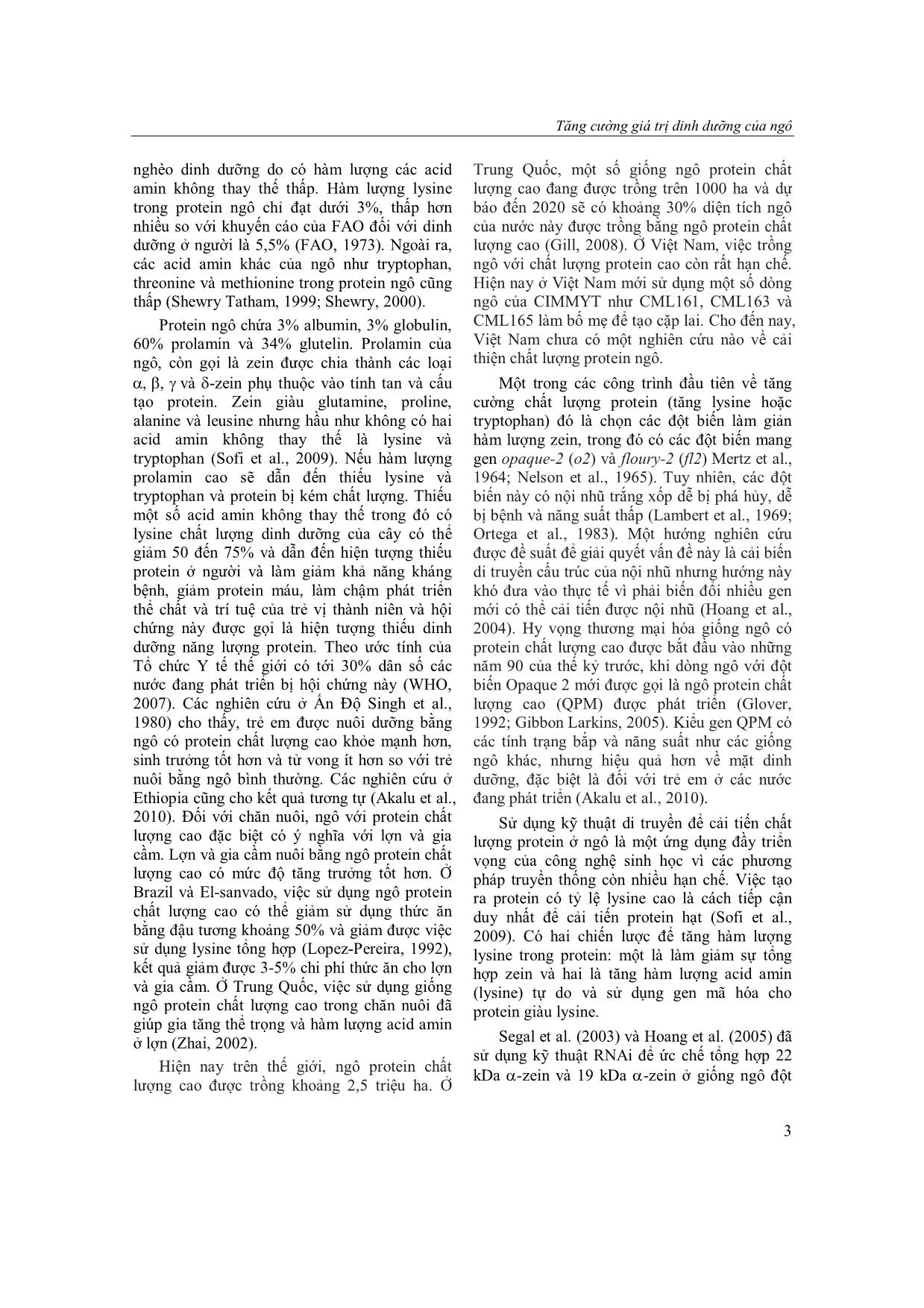
Trang 3
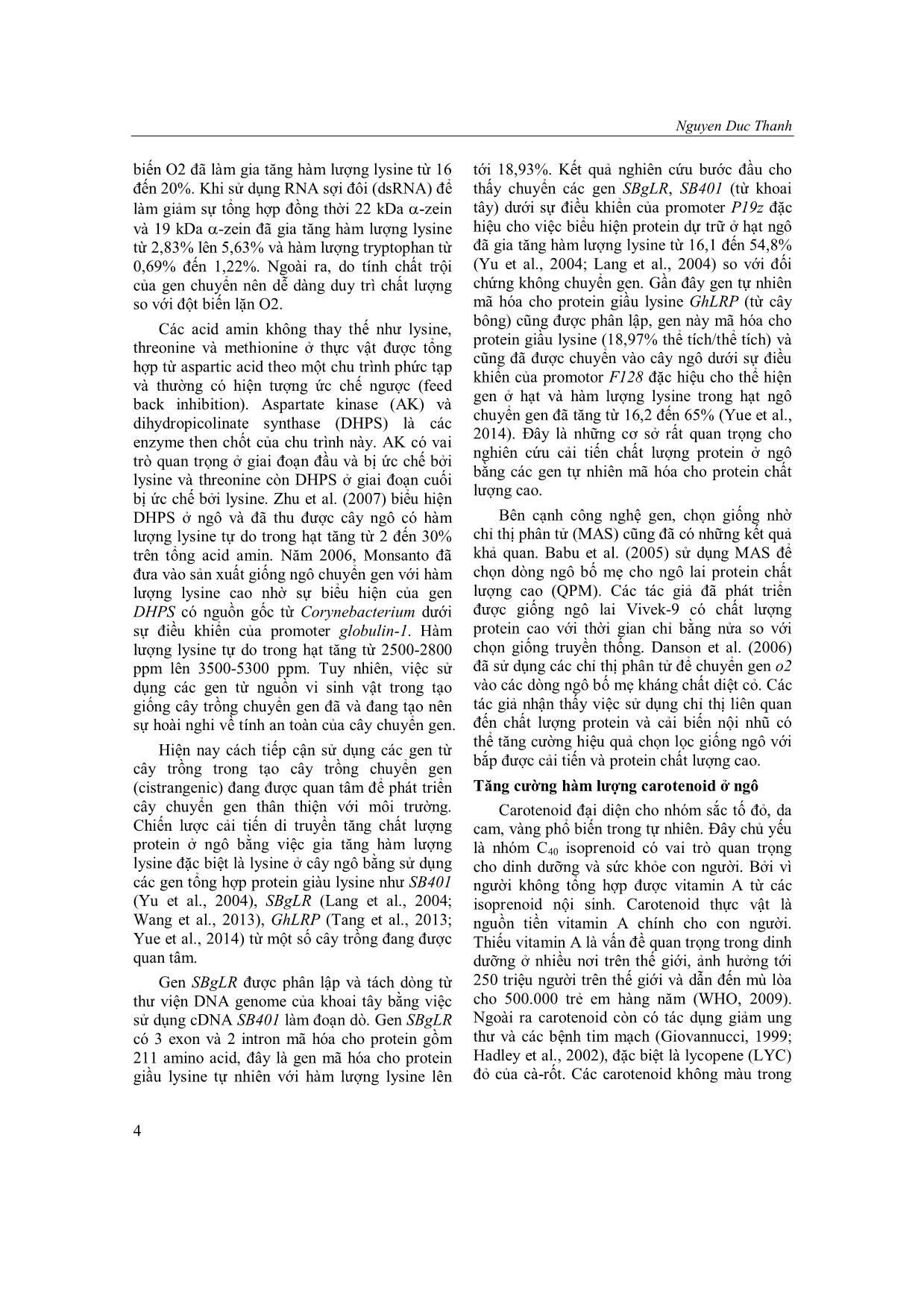
Trang 4
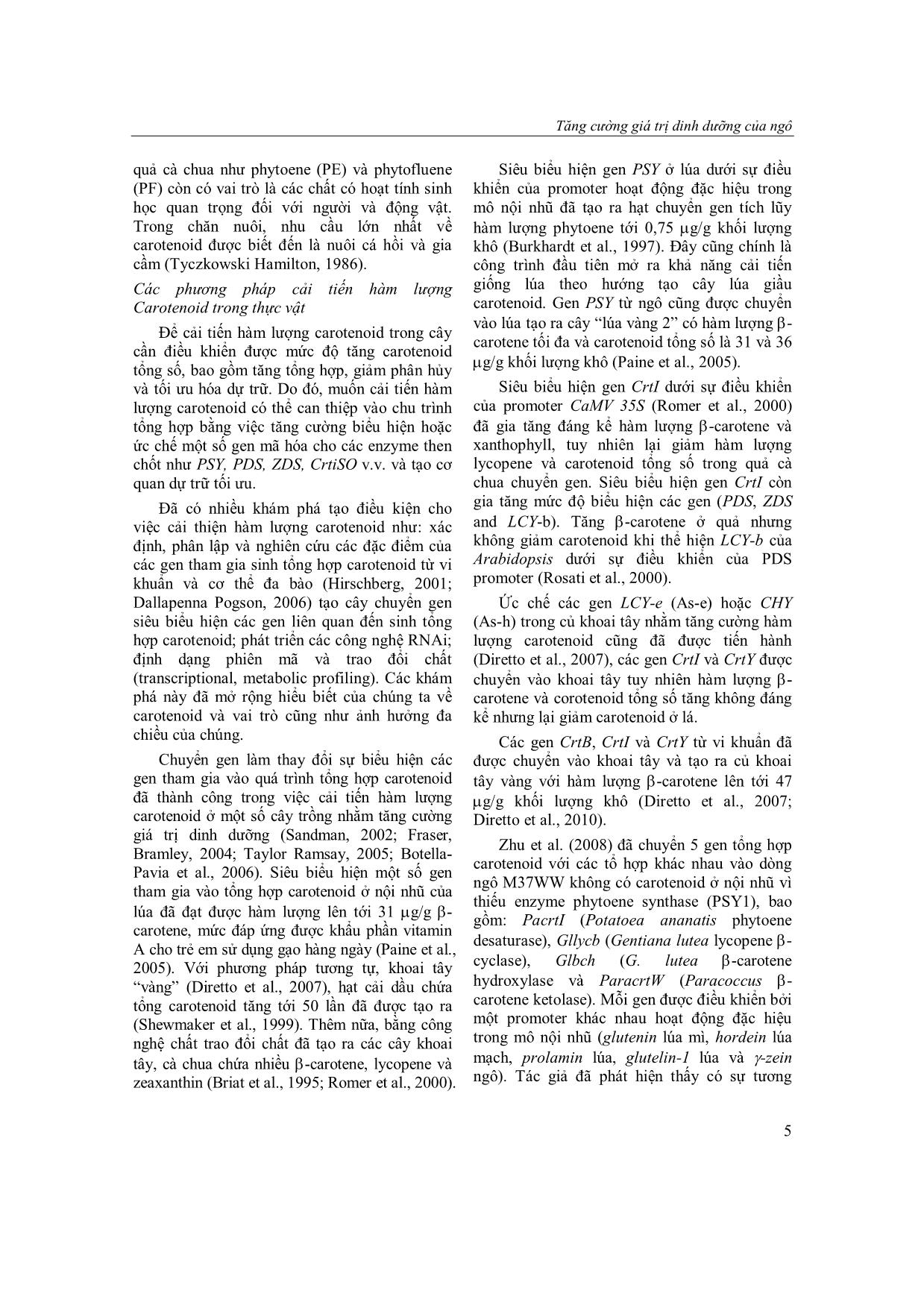
Trang 5
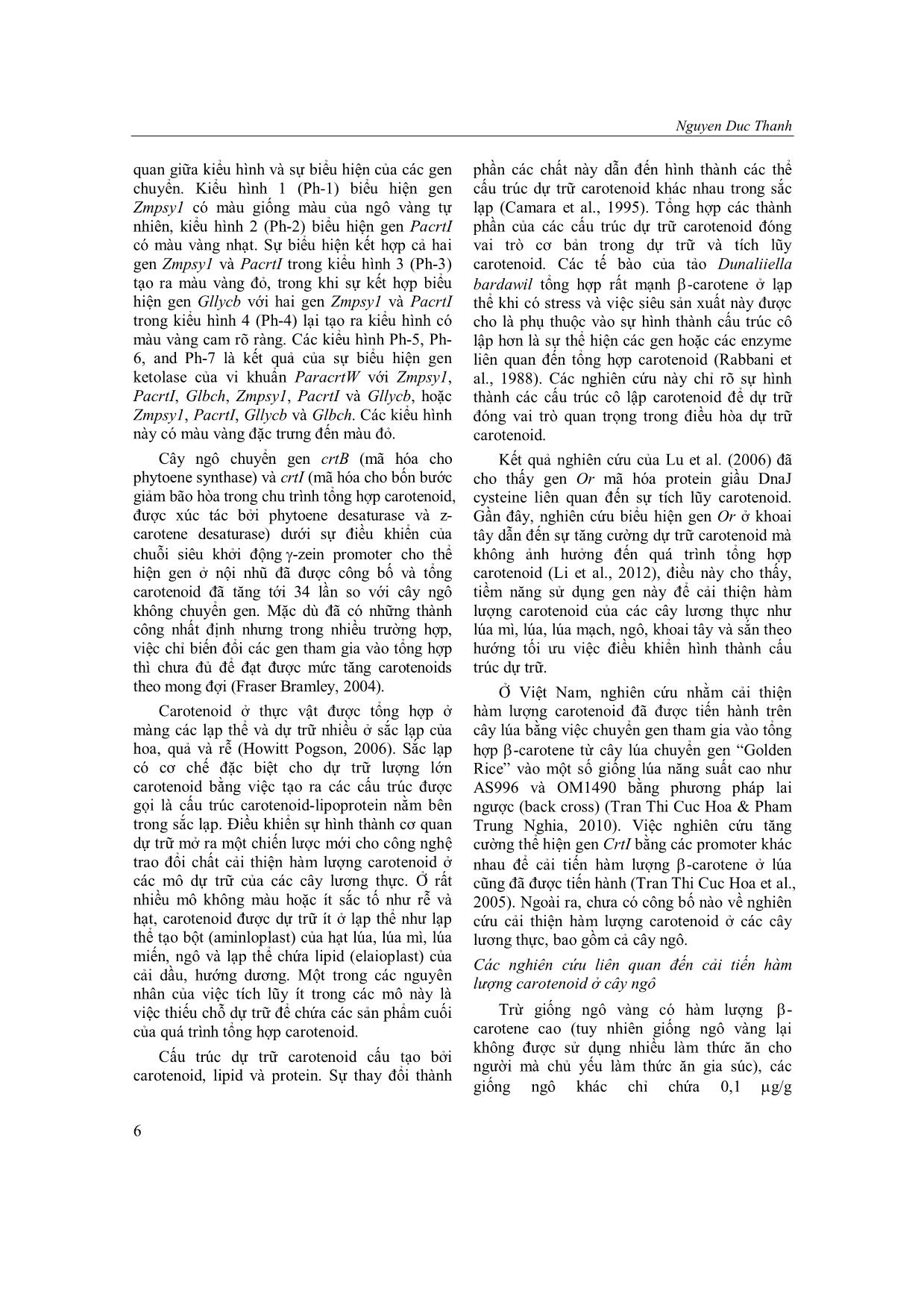
Trang 6
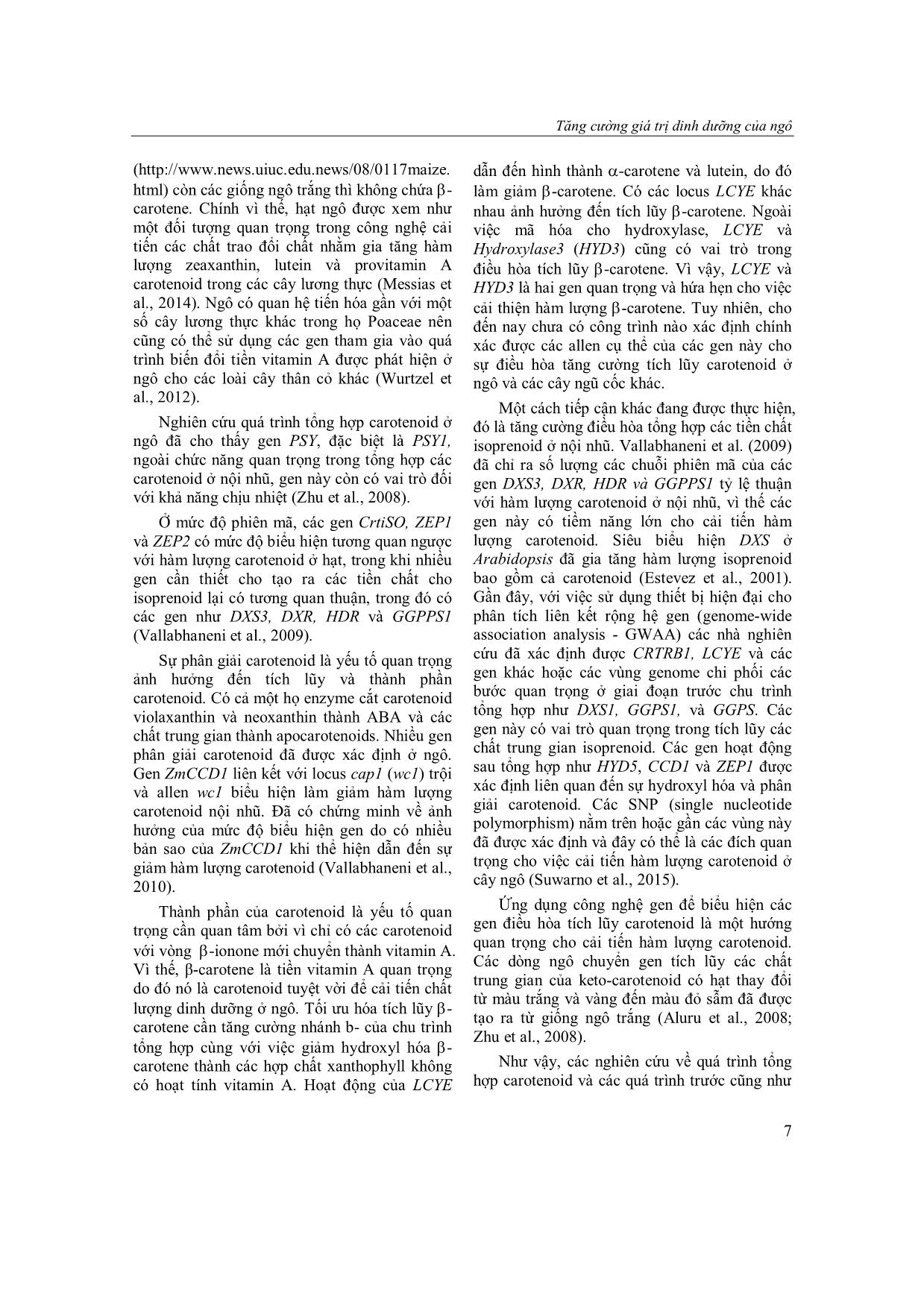
Trang 7
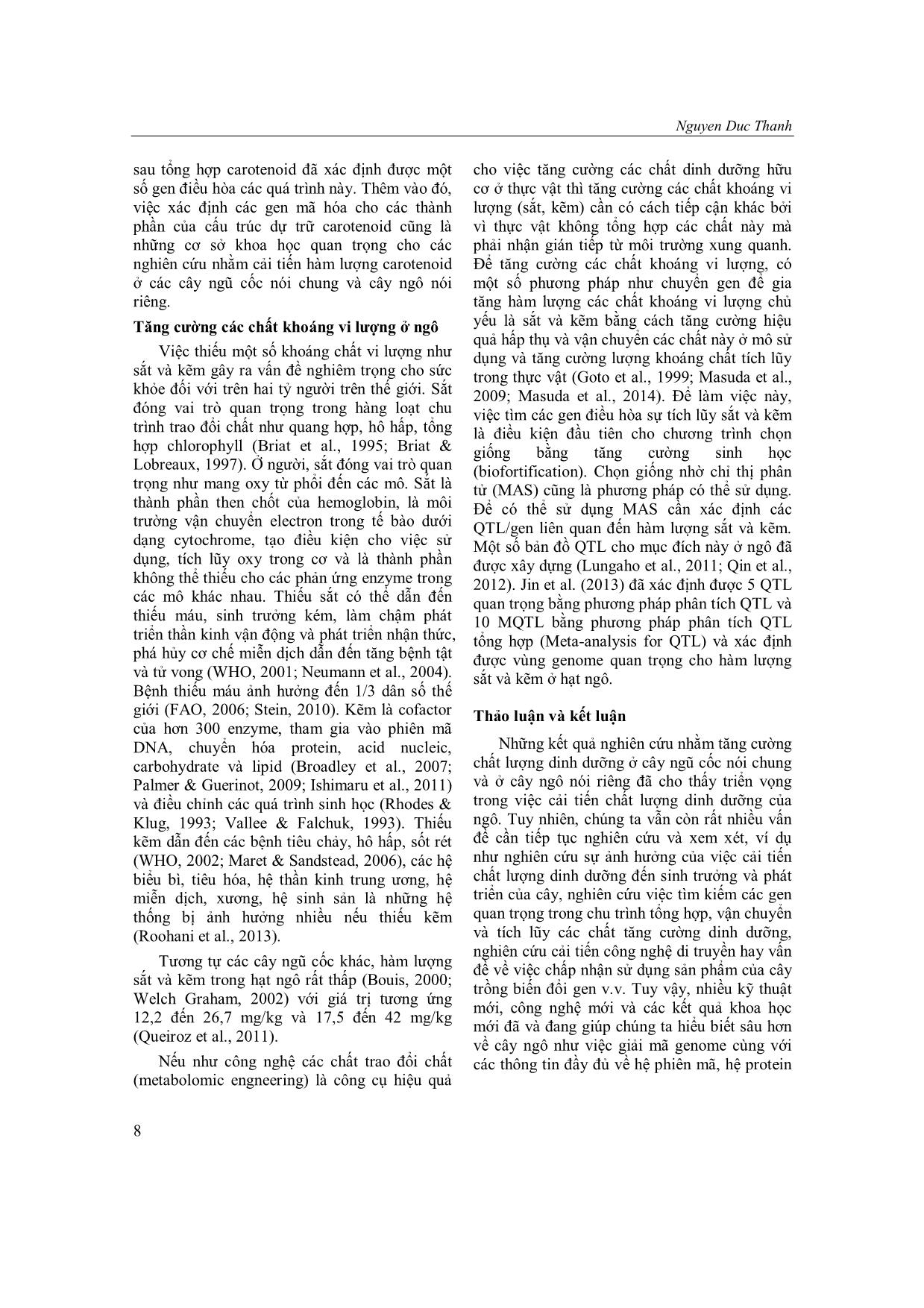
Trang 8
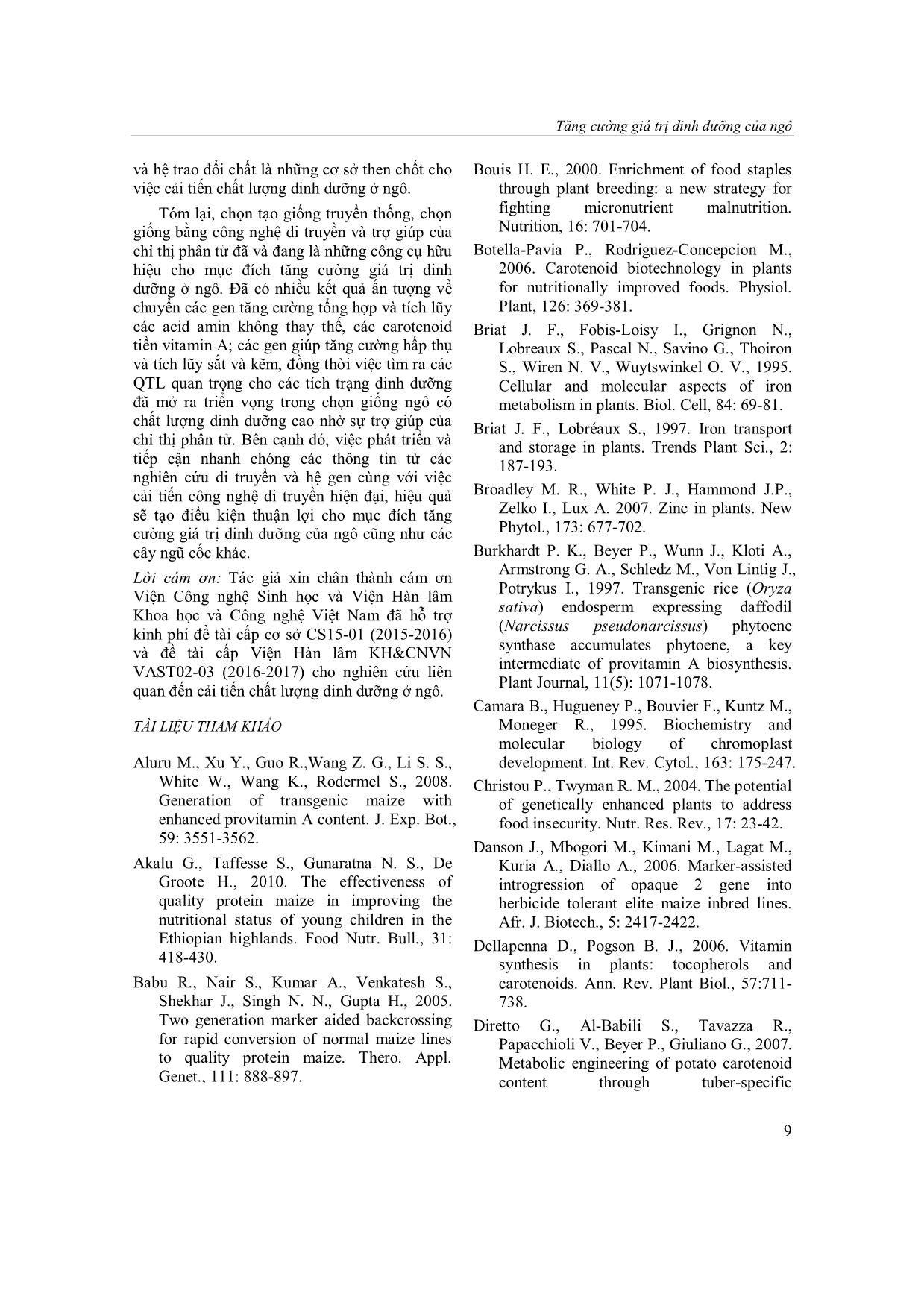
Trang 9
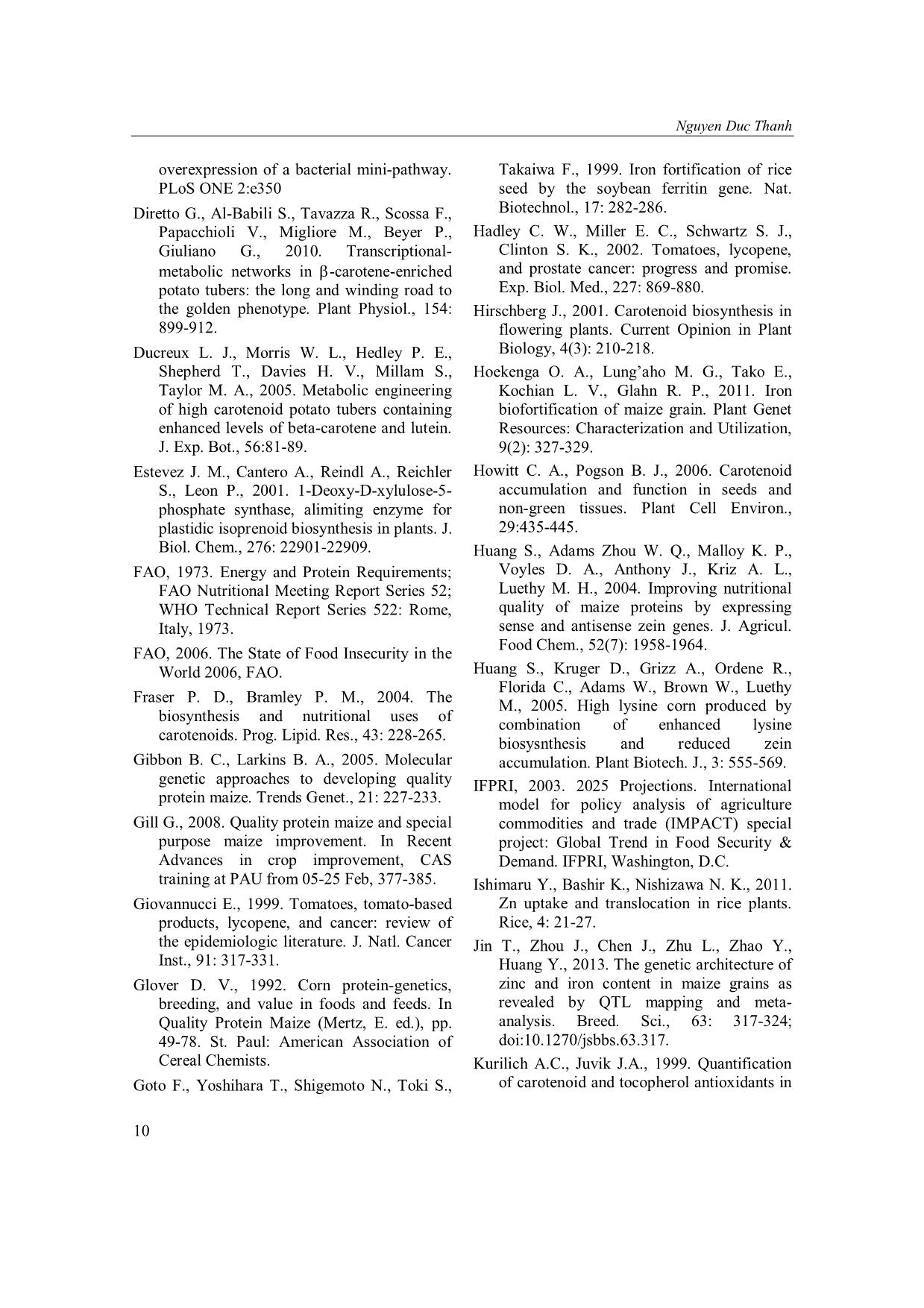
Trang 10
Tải về để xem bản đầy đủ
Tóm tắt nội dung tài liệu: Tăng cường giá trị dinh dưỡng của ngô bằng công nghệ sinh học
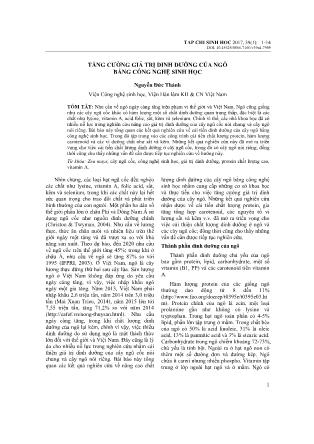
Tăng cường giá trị dinh dưỡng của ngô 1 TĂNG CƯỜNG GIÁ TRỊ DINH DƯỠNG CỦA NGÔ BẰNG CÔNG NGHỆ SINH HỌC Nguyễn Đức Thành Viện Công nghệ sinh học, Viện Hàn lâm KH & CN Việt Nam TÓM TẮT: Nhu cầu về ngô ngày càng tăng trên phạm vi thế giới và Việt Nam. Ngô cũng giống như các cây ngũ cốc khác có hàm lượng một số chất dinh dưỡng quan trọng thấp, đặc biệt là các chất như lysine, vitamin A, acid folic, sắt, kẽm và selenium. Chính vì thế, các nhà khoa học đã có nhiều nỗ lực trong nghiên cứu nâng cao giá trị dinh dưỡng của cây ngũ cốc nói chung và cây ngô nói riêng. Bài báo này tổng quan các kết quả nghiên cứu về cải tiến dinh dưỡng của cây ngô bằng công nghệ sinh học. Trong đó tập trung vào các công trình cải tiến chất lượng protein, hàm lượng carotenoid và các vi dưỡng chất như sắt và kẽm. Những kết quả nghiên cứu này đã mở ra triển vọng cho việc cải tiến chất lượng dinh dưỡng ở cây ngũ cốc, trong đó có cây ngô nói riêng; đồng thời cũng cho thấy những vấn đề cần được tiếp tục nghiên cứu về hướng này. Từ khóa: Zea mays, cây ngũ cốc, công nghệ sinh học, giá trị dinh dưỡng, protein chất lượng cao, vitamin A. Nhìn chung, các loại hạt ngũ cốc đều nghèo các chất như lysine, vitamin A, folic acid, sắt, kẽm và selenium, trong khi các chất này lại hết sức quan trọng cho trao đổi chất và phát triển bình thường của con người. Một phần ba dân số thế giới phần lớn ở châu Phi và Đông Nam Á sử dụng ngũ cốc như nguồn dinh dưỡng chính (Christou & Twyman, 2004). Nhu cầu về lương thực, thức ăn chăn nuôi và nhiên liệu trên thế giới ngày một tăng và đã vượt xa so với khả năng sản xuất. Theo dự báo, đến 2020 nhu cầu về ngũ cốc trên thế giới tăng 45%; trong khi ở châu Á, nhu cầu về ngô sẽ tăng 87% so với 1995 (IFPRI, 2003). Ở Việt Nam, ngô là cây lương thực đứng thứ hai sau cây lúa. Sản lượng ngô ở Việt Nam không đáp ứng do yêu cầu ngày càng tăng, vì vậy, việc nhập khẩu ngô ngày một gia tăng. Năm 2013, Việt Nam phải nhập khẩu 2,6 triệu tấn, năm 2014 trên 3,0 triệu tấn (Mai Xuan Trieu, 2014), năm 2015 lên tới 7,55 triệu tấn, tăng 71,2% so với năm 2014 ( Nhu cầu ngày càng tăng, trong khi chất lượng dinh dưỡng của ngô lại kém, chính vì vậy, việc thiếu dinh dưỡng do sử dụng ngô là một thách thức lớn đối với thế giới và Việt Nam. Đây cũng là lý do cho nhiều nỗ lực trong nghiên cứu nhằm cải thiện giá trị dinh dưỡng của cây ngũ cốc nói chung và cây ngô nói riêng. Bài báo này tổng quan các kết quả nghiên cứu về nâng cao chất lượng dinh dưỡng của cây ngô bằng công nghệ sinh học nhằm cung cấp những cơ sở khoa học và thực tiễn cho việc tăng cường giá trị dinh dưỡng của cây ngô. Những kết quả nghiên cứu nhận được về cải tiến chất lượng protein, gia tăng tổng hợp carotenoid, các nguyên tố vi lượng sắt và kẽm v.v. đã mở ra triển vọng cho việc cải thiện chất lượng dinh dưỡng ở ngô và các cây ngũ cốc; đồng thời cũng cho thấy những vấn đề cần được tiếp tục nghiên cứu. Thành phần dinh dưỡng của ngô Thành phần dinh dưỡng chủ yếu của ngô bao gồm protein, lipid, carbonhydrate, một số vitamin (B1, PP) và các carotenoid tiền vitamin A. Hàm lượng protein của các giống ngô thường dao động từ 8 đến 11% ( m). Protein chính của ngô là zein, một loại prolamine gần như không có lysine và tryptophan. Trong hạt ngô toàn phần có 4-5% lipid, phần lớn tập trung ở mầm. Trong chất béo của ngô có 50% là acid linoleic, 31% là oleic acid, 13% là panmitic acid và 3% là stearic acid. Carbonhydrate trong ngô chiếm khoảng 72-73%, chủ yếu là tinh bột. Ngoài ra ở hạt ngô non có thêm một số đường đơn và đường kép. Ngô chứa ít canxi nhưng nhiều phospho. Vitamin tập trung ở lớp ngoài hạt ngô và ở mầm. Ngô có TAP CHI SINH HOC 2017, 39(1): 1-14 DOI: 10.15625/0866-7160/v39n1.7909 Nguyen Duc Thanh 2 nhiều vitamin B1 nhưng vitamin PP thấp cộng với việc thiếu tryptophan-một tiền chất để tạo vitamin PP. Vì vậy, nếu ăn ngô đơn thuần và kéo dài sẽ mắc bệnh Pellagra (bệnh thiếu vitamin PP). Hàm lượng carotenoid tiền vitamin A ở ngô rất thấp. Trong hạt ngô chứa chủ yếu các dạng carotenoid tiền vitamin A gồm -carotene, - carotene và -cryptoxanthin nhưng hàm lượng thấp với giá trị tương ứng từ 0,0-1,3; 0,13-2,7 và 0,13-1,9 nmol/g (Kurilich Juvik, 1999). Hiện nay, nhờ sự cố gắng trong nghiên cứu cải tạo giống, các nhà khoa học đã tạo được giống ngô có hàm lượng -carotene tổng số đạt 15 g/g so với 0,1 g/g ở giống ngô bình thường ( html). Tuy nhiên, hàm lượng này vẫn rất thấp khi so sánh với carrot (83,32 g/g) ( ural-food-sources-of-beta-carotene.php. Hàm lượng các chất vi lượng như sắt và kẽm ở ngô cũng thấp (Bouis, 2000; Welch Graham, 2002). Hàm lượng kẽm trung bình trong hạt ngô là 2,0 mg/100 g hạt (Ortiz- Monasterio et al., 2007) còn hàm lượng sắt chỉ từ 1,2 đến 3,6 mg/100 g (Queiroz et al., 2011). Do thiếu lysine, triptophan, ít tiền vitamin A, kẽm và sắt nên chất lượng dinh dưỡng của n ... d., 227: 869-880. Hirschberg J., 2001. Carotenoid biosynthesis in flowering plants. Current Opinion in Plant Biology, 4(3): 210-218. Hoekenga O. A., Lung’aho M. G., Tako E., Kochian L. V., Glahn R. P., 2011. Iron biofortification of maize grain. Plant Genet Resources: Characterization and Utilization, 9(2): 327-329. Howitt C. A., Pogson B. J., 2006. Carotenoid accumulation and function in seeds and non-green tissues. Plant Cell Environ., 29:435-445. Huang S., Adams Zhou W. Q., Malloy K. P., Voyles D. A., Anthony J., Kriz A. L., Luethy M. H., 2004. Improving nutritional quality of maize proteins by expressing sense and antisense zein genes. J. Agricul. Food Chem., 52(7): 1958-1964. Huang S., Kruger D., Grizz A., Ordene R., Florida C., Adams W., Brown W., Luethy M., 2005. High lysine corn produced by combination of enhanced lysine biosysnthesis and reduced zein accumulation. Plant Biotech. J., 3: 555-569. IFPRI, 2003. 2025 Projections. International model for policy analysis of agriculture commodities and trade (IMPACT) special project: Global Trend in Food Security & Demand. IFPRI, Washington, D.C. Ishimaru Y., Bashir K., Nishizawa N. K., 2011. Zn uptake and translocation in rice plants. Rice, 4: 21-27. Jin T., Zhou J., Chen J., Zhu L., Zhao Y., Huang Y., 2013. The genetic architecture of zinc and iron content in maize grains as revealed by QTL mapping and meta- analysis. Breed. Sci., 63: 317-324; doi:10.1270/jsbbs.63.317. Kurilich A.C., Juvik J.A., 1999. Quantification of carotenoid and tocopherol antioxidants in Tăng cường giá trị dinh dưỡng của ngô 11 Zea mays. J. Agric. Food. Chem., 47:1948- 55. Lang Z., Zhao Q., Yu J., Zhu D., Ao G., 2004. Cloning of potato SBgLR gene and its intron splicing in transgenic maize. Plant Sci., 166: 1227-1233. Lambert R., Alexander D., Dudley J., 1969. Relative performance of normal and modified protein (opaque-2) maize hybrids. Crop Sci., 9: 242-243. Li L., Yang Y., Xu Q., Owsiany K., Welsch R., Chitchumroonchokchai C., Lu S., Van Eck J., Deng X. X., Failla M., Thannhauser T. W., 2012. The Or gene enhances carotenoid accumulation and stability during post- harvest storage of potato tubers. Mol. Plant, 5(2): 339-352. Lopez-Pereira M. A., 1992. The economics of quality protein maize fully acknowledged as an animal feed: case studies of Brazil and El Salvador. CIMMYT Economics Working Paper 92-06. CIMMYT, Mexico, D.F. Lu S., Eck Van J., Zhou X., Lopez A.B., O’Halloran D. M., Cosman K. M., Conlin B. J., Paolillo D. J., Garvin D. F., Vrebalov J., Kochian L. V., Kupper H., Earle E. D., Cao J., Li L., 2006. The cauliflower Or gene encodes a DnaJ cysteine-ricdomain containing protein that mediates high levels of beta-carotene accumulation. Plant Cell, 18: 3594-3605. Lung’aho M. G., Mwaniki A. M., Szalma S. J., Hart J. J., Rutzke M. A., Kochian L. V., Glahn R. P., Hoekenga O. A., 2011. Genetic and physiological analysis of iron biofortification in maize kernels. PLoS One 6: e20429. doi: 10.1371/journal.pone. 0020429. Lyons G. H., Stangoulis J., Graham R. D., 2004. Exploiting micronutrient interaction to optimize biofortification programs: The case for inclusion of selenium and iodine in the Harvest Plus Program. Nutr. Rev., 62: 247- 252. Mai Xuan Trieu, 2014. Maize production in Vietnam. 12 Maize conference and expert consultation on “Maize for food, feed, nutrition and environmental security” 30 October-01 November, 2014, Bangkok, Thailand. Extended Summaries. pp. 332- 338. Maret W., Sandstead H. H., 2006. Zinc requirements and the risks and benefits of zinc supplementation. J. Trace Elem. Med. Biol., 20: 3-18. Masuda H., Usuda K., Kobayashi T., Ishimaru Y., Kakei Y., Takahashi M., Higuchi K., Nakanishi H., Mori S., Naoko K. Nishizawa N. K., 2009. Overexpression of the barley nicotianamine synthase gene HvNAS1 increase iron and zinc concentrations in rice grains. Rice, 2: 155-166. Masuda H., Ishimaru Y., Aung M. S., Kobayashi T., Kakei Y., Takahashi M., Higuchi K., Nakanishi H., Nishizawa N. K., 2012. Iron biofortification in rice by the introduction of multiple genes involved in iron nutrition. Sci. Report 2: 543 DOI: 10.1038/srep00543. Messias R. S., Galli V., dos Anjose Silva S. D., Rombaldi C. V., 2014 Carotenoid biosynthetic and catabolic pathways: Gene expression and carotenoid content in grains of maize landraces. Nutrients, 6: 546-563. Mertz E. T., Bates L. S., Nelson E. Z., 1964. Mutant gene that changes protein composition and increases lysine content of maize endosperm. Science, 145: 279-280. Nelson E. Z., Mertz E. T., Bates L. S., 1965. Second mutant gene affecting the amino acid pattern of maize endosperm proteins. Science, 150: 1469-1470. Neumann C. G., Gewa C., Bwibo N. O., 2004. Child nutrition in developing countries. Pediatr Ann, 33(10): 658-674. Ortega E. I., Bates L. S., 1983. Biochemical and agronomic studies of two modified hard- endosperm opaque-2 maize (Zea mays L.) populations. Cereal Chem., 60: 107-111. Ortiz-Monasterio J. I., Palacios-Rojas N., Meng E., Pixley K., Trethowan R., Pena R. J., 2007. Enhancing the mineral and vitamin content of wheat and maize through plant breeding. J. Cereal Sci., 46:293-307. Nguyen Duc Thanh 12 Paine J. A., Shipton C. A., Chaggar S., Howells R. M., Kennedy M. J., Vernon G., Wright S. Y., Hinchliffe E., Adams J. L., Silverstone A. L., Drake R., 2005. Improving the nutritional value of Golden Rice through increased pro-vitamin A content. Nat. Biotechnol., 23: 482-487. Palmer C. M., Guerinot M. L., 2009. Facing the challenges of Cu, Fe and Zn homeostasis in plants. Nat. Chem. Biol., 5: 333-340. Queiroz V. A. V., de Oliveira Guimaraes P. E., Queiroz L. R., de Oliveira Guedes E., Vasconcelos V. D. B., Guimaras L. J., de Aquino Ribeiro P. E., Schaffert R. E., 2011. Iron and zinc availability in maize lines. Ciênc. Tecnol. Aliment., Campinas, 31(3): 577-583. Qin H. N., Cai Y. L., Liu Z. Z., Wang G. Q., Wang J. G., Guo Y. Wang H., 2012. Identification of QTL for zinc and iron concentration in maize kernel and cob. Euphytica, 187: 345-358. Rabbani S., Beyer P., Lintig J., Hugueney P., Kleinig H., 1998. Induced beta-carotene synthesis driven by triacylglycerol deposition in the unicellular alga Dunaliella bardawil. Plant Physiol., 116: 1239-1248. Rhodes D., Klug A., 1993. Zinc fingers. Sci. Am., 268: 56-65. Romer S., Fraser P. D., Kiano J. W., Shipton C. A., Misawa N., Schuch W., Bramley P. M., 2000. Elevation of the provitamin A content of transgenic tomato plants. Nat. Biotechnol., 18: 666-669. Rosati C., Aquilani R., Dharmapuri S., Pallara P., Marusic C., Tavazza R., Bouvier F., Camara B., Guiliano G., 2000. Metabolic engineering of beta-carotene and lycopene content in tomato fruit. Plant Journal, 24(3): 413-420. Roohani N., Hurrell R., Kelishadi R., Schulin R., 2013. Zinc and its importance for human health: An integrative review. Journal of Research in Medical Sciences: The Official Journal of Isfahan University of Medical Sciences, 18(2):144-157. Saltzman A., Birol E., Bouis H. E., Boy E., De Moura F. F., Islam Y., Pfeiffer W. H., 2013. Biofortification: Progress toward a more nourishing future. Global Food Security, 2: 9-17. Sandmann G., 2002. Molecular evolution of carotenoid biosynthesis from bacteria to plants. Physiol. Plant, 116: 431-440. Segal G., Songh R., Messing J., 2003. A new opaque variant of maize by single dominant RNA-I inducing transgene. Genet., 165: 387-397. Shewmaker C. K., Sheehy J. A., Daley M., Colburn S., Ke D. Y., 1999. Seed-specific overexpression of phytoene synthase: increase in carotenoids and other metabolic effects. Plant J., 20: 401-412. Shewry P. R., Tatham A. S., 1999. The characteristics, structures and evolutionary relationships of prolamins. In Seed Proteins; Shewry, P. R., Casey, R., Eds.; Kluwer Academic Publishers: Dordrecht, The Netherlands, pp 11-33. Shewry P. R., 2000. Seed proteins. In Seed Technology and its Biological Basis; Black, M., Bewley, J. D., Eds.; CRC Press: Boca Raton, FL, 2000; pp 42-84. Singh J., Koshy S., Agarwal K., Singh N. N., Lodha M., Sethi A., 1980. Relative efficiency of opaque-2 maize in growth of preschool children. Ind. J. Nutr. Dietet., 17: 326-334. Sofi P. A., Wani S. A., Rather A. G., Wani S. H., 2009. Review article: Quality protein maize (QPM): Genetic manipulation for the nutritional fortification of maize. J. Plant Breed. Crop Sci., 1(6): 244-253. Stein A. J., 2010. Global impacts of human mineral malnutrition. Plant Soil, 335: 133- 154. Suwarno W. B., Pixley K. V., Palacios-Rojas N., Kaeppler S. M., Babu R., 2015. Genome-wide association analysis reveals new targets for carotenoid biofortification in maize. Theor. Appl. Genet., 128: 851-864. Tako E., Hoekenga O. A., Kochian L. V., Glahn R. P., 2013. High bioavailablilty iron maize Tăng cường giá trị dinh dưỡng của ngô 13 (Zea mays L.) developed through molecular breeding provides more absorbable iron in vitro (Caco-2 model) and in vivo (Gallus gallus). Nutrition Journal, 12: 3. doi:10.1186/1475-2891-12-3 Tang M., He X., Luo Y., Ma L., Tang X., Huang K., 2013. Nutritional assessment of transgenic lysine-rich maize compared with conventional quality protein maize. J. Sci. Food Agric., 93: 1049-1054. Taylor M., Ramsay G., 2005. Carotenoid biosynthesis in plant storage organs: recent advances and prospects for improving plant food quality. Physiol. Plant, 124:143-151. Tran Thi Cuc Hoa, Al-Babili S., Schaub P., Potrykus I., Beyer P., 2005. Approach to improve CrtI expression in rice endosperm for increasing the -carotene in Gold Rice. Omonrice, 13: 1-11. Tran Thi Cuc Hoa, Pham Trung Nghia, 2010. Expression of -Carotene in advanced back crosses of the high-yield rice varieties to the transgenic gold rice lines. Omonrice, 17: 1- 7. Tyczkowski J. K., Hamilton P. B., 1986. Absorption, transport, and deposition in chickens of lutein diester, a carotenoid extracted from marigold (Tagetes erecta) petals. Poultry Sci., 65: 1526-1531. Vallabhaneni R., Gallagher C. E., Lic ciardello N., Cuttriss A. J., Quinlan R. F., Wurtzel E. T., 2009. Metabolitesorting of a germplasm collection reveals the Hydroxylase3 locus as a new target for maize provitamin A biofortification. Plant Physiol., 151:1635- 1645. Vallabhaneni R., Bradbury L. M. T., Wurtzel E. T., 2010.The carotenoid dioxygenase gene family in maize, sorghum, and rice. Arch. Biochem. Biophys., 504: 104-111. Vallee B. L., Falchuk K. H., 1993. The biochemical basis of zinc physiology. Physiol. Rev., 73: 79-118. Velu G., Ortiz-Monasterio I., Cakmak I., Hao Y., Singh R. P., 2014. Biofortification strategies to increase grain zinc and iron concentrations in wheat. J. Cereal Sc., 59(3): 365-372. Wang M., Liu C., Li S., Zhu D., Zhao Q., Yu J., 2013. Improved nutritive quality and salt resistance in transgenic maize by simultaneously overexpression of a natural Lysine-rich protein gene, SBgLR, and an ERF transcription factor gene, TSRF1. Int. J. Mol. Sci., 14: 9459-9474. Welch R. M., Graham R. D., 2002. Breeding crops for enhanced micronutrient content. Plant Soil, 245: 205-214. White P. J., Broadley M. R., 2005. Biofortifying crops with essential mineral elements. Trends Plant Sci., 10: 586-593. WHO, 2002. World Health Report 2002. http:// www.who.int/whr/2002/en/. WHO, 2001. Iron deficiency anemia assessment, prevention and control. A guide for program managers. Geneva: WHO/NDH: 15-21. WHO, 2007. Protein and amino acid requirements in human nutrition. In: Report of a joint WHO/FAO/UNU experts conclu. Geneva: Switzerland WHO Press, RS_935_eng.pdf. WHO, 2009. Global prevalence of vitaminA deficiency in populations at risk 1995-2005. In: WHO Global Database on Vitamin A Deficiency, pp 1-55. Wurtzel T. E., Cuttriss A., Vallabhaneni R., 2012. Maize provitamin A carotenoids, current resources, and future metabolic engineering challenges. Fronties in Plant Sci., 3:1-12, doi: 10.3389/fpls.2012.00029. Yu J., Peng P., Zhang X., Zhao Q., Zhu D., Sun X., Liu J., Ao G., 2004. Seed-specific expression of the lysine-rich protein gene sb401 significantly increases both lysine and total protein content in maize seeds. Mol. Breed., 14: 1-7. Yue J., Li C., Zhao Q., Zhu D., Yu J., 2014. Seed-specific expression of a Lysine-rich protein gene, GhLRP, from cotton significantly increases the Lysine content in maize seeds. Int. J. Mol. Sci., 15: 5350-5365. doi:10.3390/ijms15045350. Nguyen Duc Thanh 14 Zhai S., 2002. Nutritional evaluation and utilization of QPM Zhang Dan 9404 in laying ren feeds. M.Sc. Thesis. North Western Agri. & Forestry Univ. of Sci. & Tech, China, 13-21. Zhu C., Naqvi S., Sonia G., Pelacho A., Capell T., Christou P., 2007. Transgenic strategies for nutritional enhancement of plant. Trends Plant Sci 12: 548-555. Zhu C. F., Naqvi S., Breitenbach J., Sandmann G., Christou P., Capell T., 2008. Combinatorial genetic transformation generates a library of metabolic phenotypes for the carotenoid pathway in maize. Proc. Natl. Acad. Sci. USA 105: 18232-18237. m. ral-food-sources-of-beta-carotene.php. html. THE ENHANCEMENT OF NUTRITION VALUE OF MAIZE BY BIOTECHNOLOGY Nguyen Duc Thanh Institute of Biotechnology, VAST SUMMARY The demand on maize is increasing both in the world and Vietnam. While, as other cereals, maize is low in important nutrition compounds, especially, lysine, vitamin A, folic acid, iron, zinc and selenium. Therefore, there have been many attempts in the researches on improvement of nutrition value of cereal crops, in general, and maize, in particular. This article reviews the published results obtained from the studies aimed to enhance maize nutrition value by biotechnological tools. Especially, the progress in the improvement of protein quality, carotenoid content and micro-nutrition elements such as ferrous and zinc will be highlighted. The published results showed bright prospect for the improvement of nutrition value of maize and other cereal crops as well as the issues that need the further investigation. Keywords: Zea mays, Biotechnology, cereals, nutrition value, high quality protein, vitamin A. Citation: Nguyen Duc Thanh, 2017. The enhancement of nutrition value of maize by biotechnology. Tap chi Sinh hoc, 39(1): 1-14. DOI: 10.15625/0866-7160/v39n1.7909. *Corresponding author: nguyenducthanh_pcg@ibt.ac.vn. Received 17 March 2016, accepted 20 March 2017
File đính kèm:
tang_cuong_gia_tri_dinh_duong_cua_ngo_bang_cong_nghe_sinh_ho.pdf