Morpho-Kinematics of the molecular gas in a quasar host galaxy at redshift z = 0:654
We present a new study of archival ALMA observations of the CO(2-1) line emission
of the host galaxy of quasar RX J1131 at redshift z=0.654, lensed by a foreground galaxy. A
simple lens model is shown to well reproduce the optical images obtained by the Hubble Space
Telescope. Clear evidence for rotation of the gas contained in the galaxy is obtained and a simple
rotating disc model is shown to give an excellent overall description of the morpho-kinematics of
the source. The possible presence of a companion galaxy suggested by some previous authors is
not confirmed. Detailed comparison between model and observations gives evidence for a more
complex dynamics than implied by the model. Doppler velocity dispersion within the beam size in
the image plane is found to account for the observed line width.
Keywords: galaxies: evolution – galaxies: ISM – radio lines: galaxies.
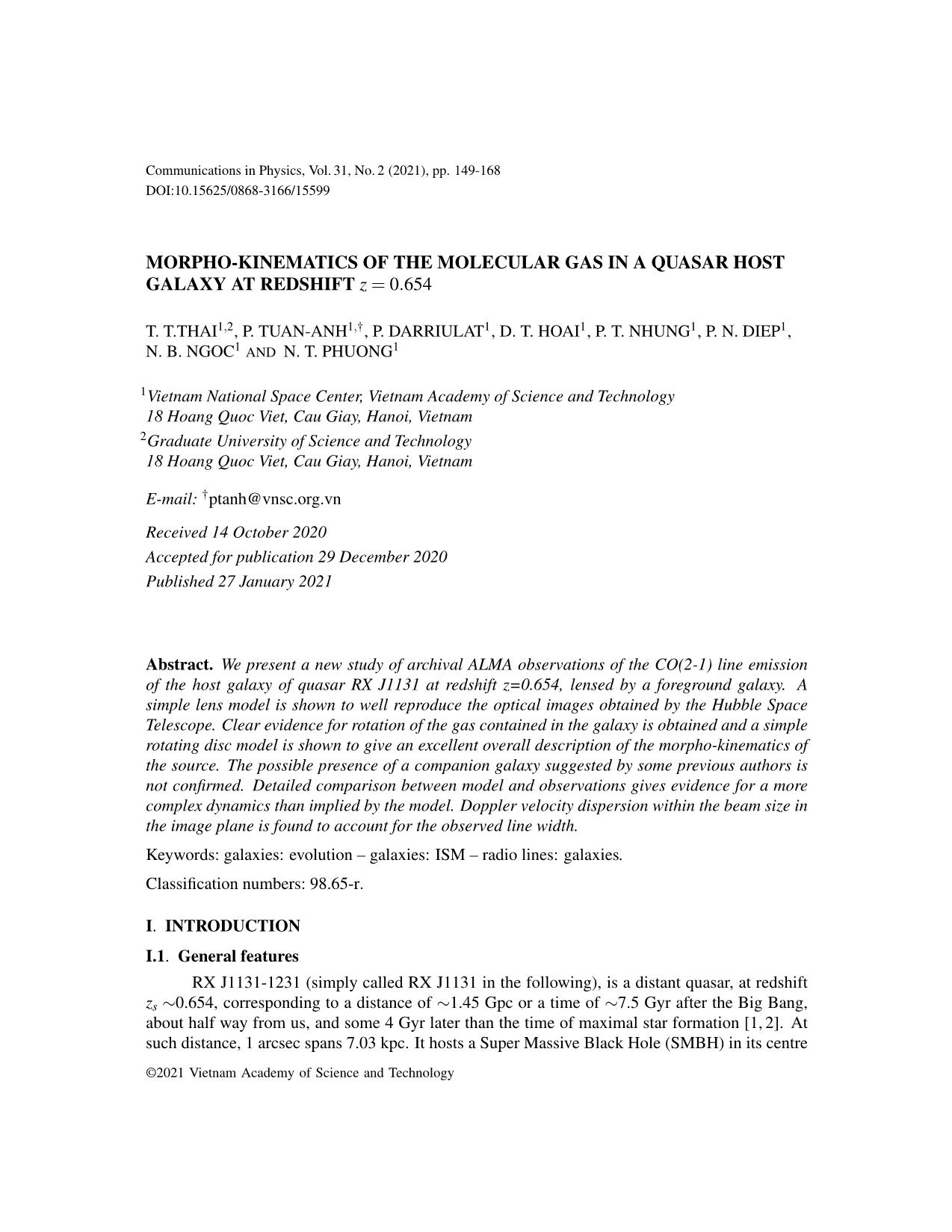
Trang 1
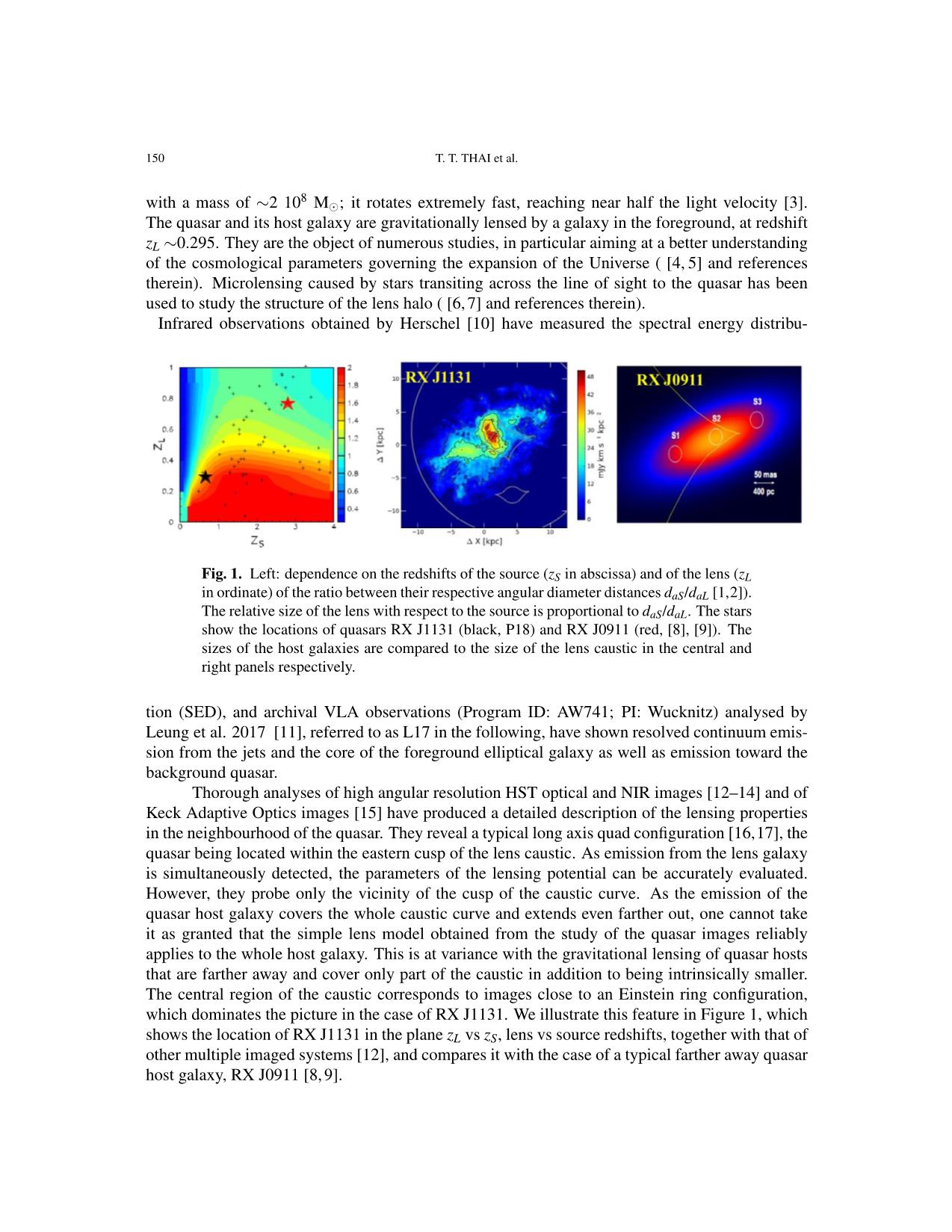
Trang 2
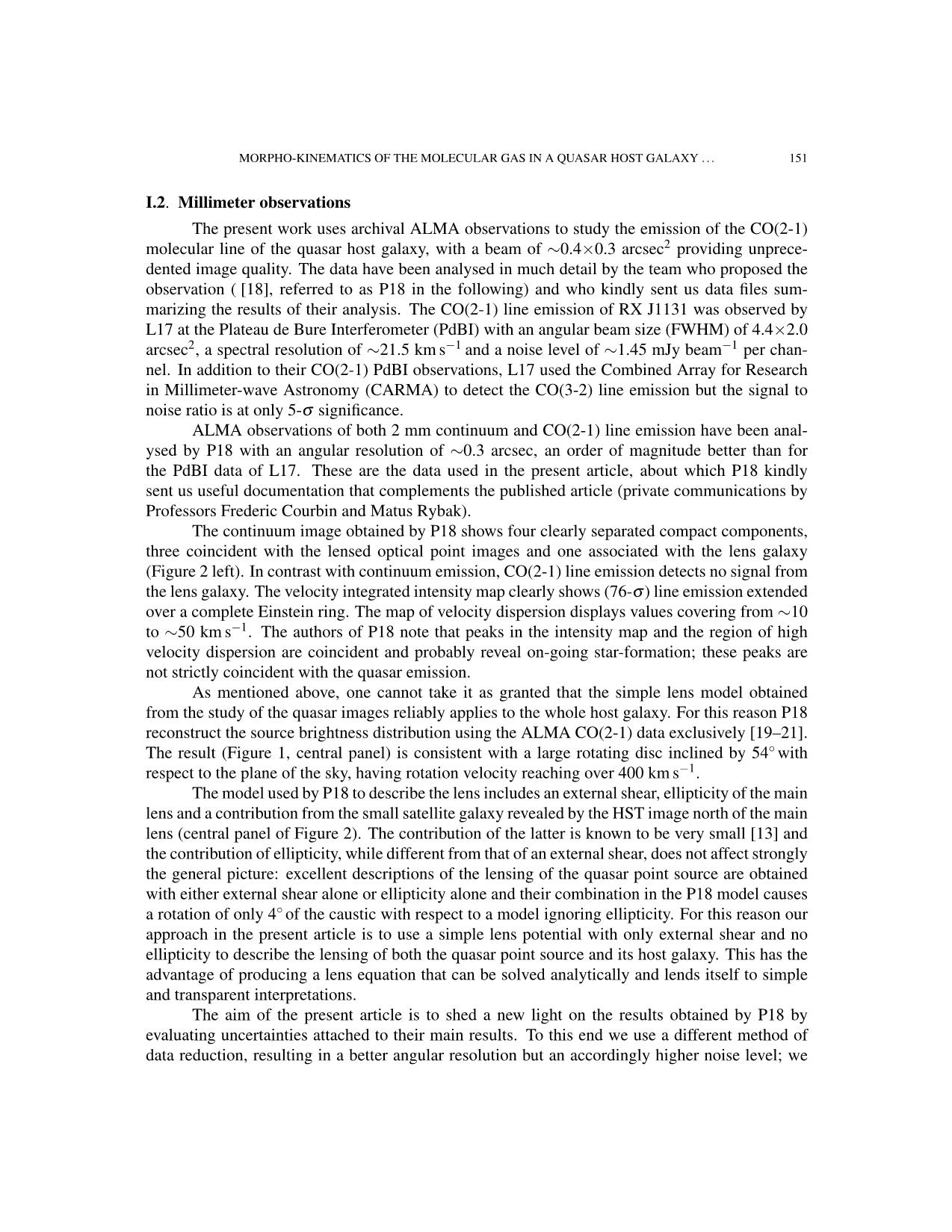
Trang 3
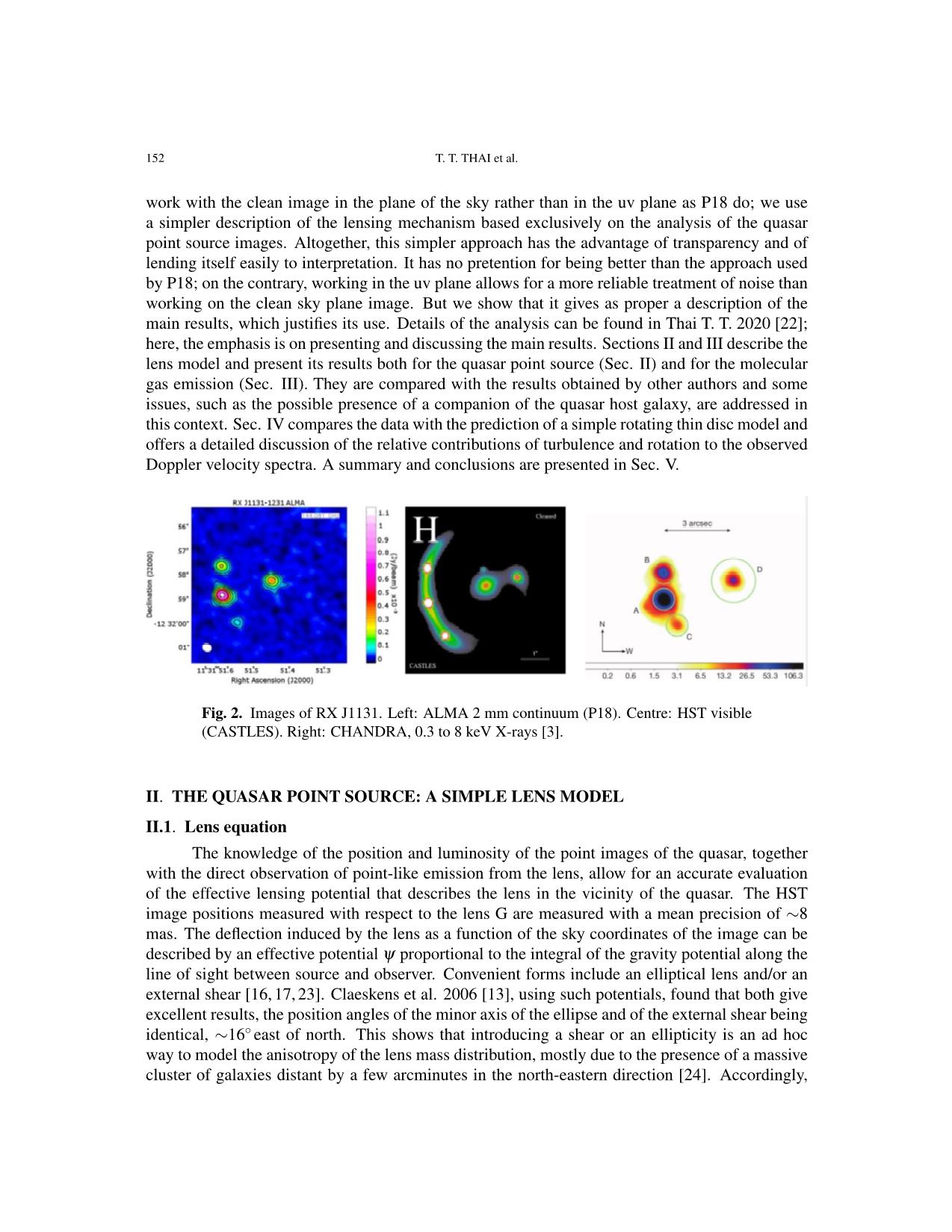
Trang 4
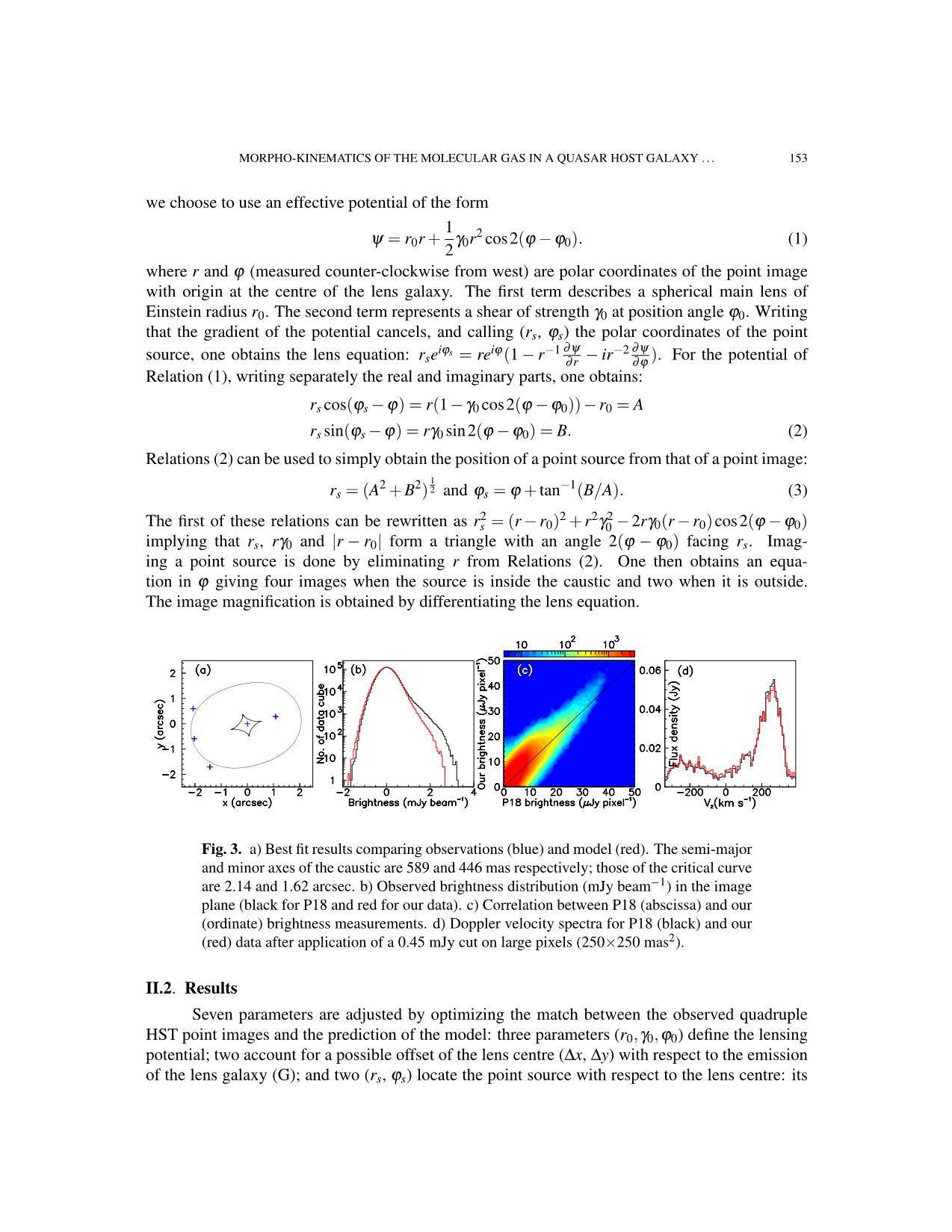
Trang 5
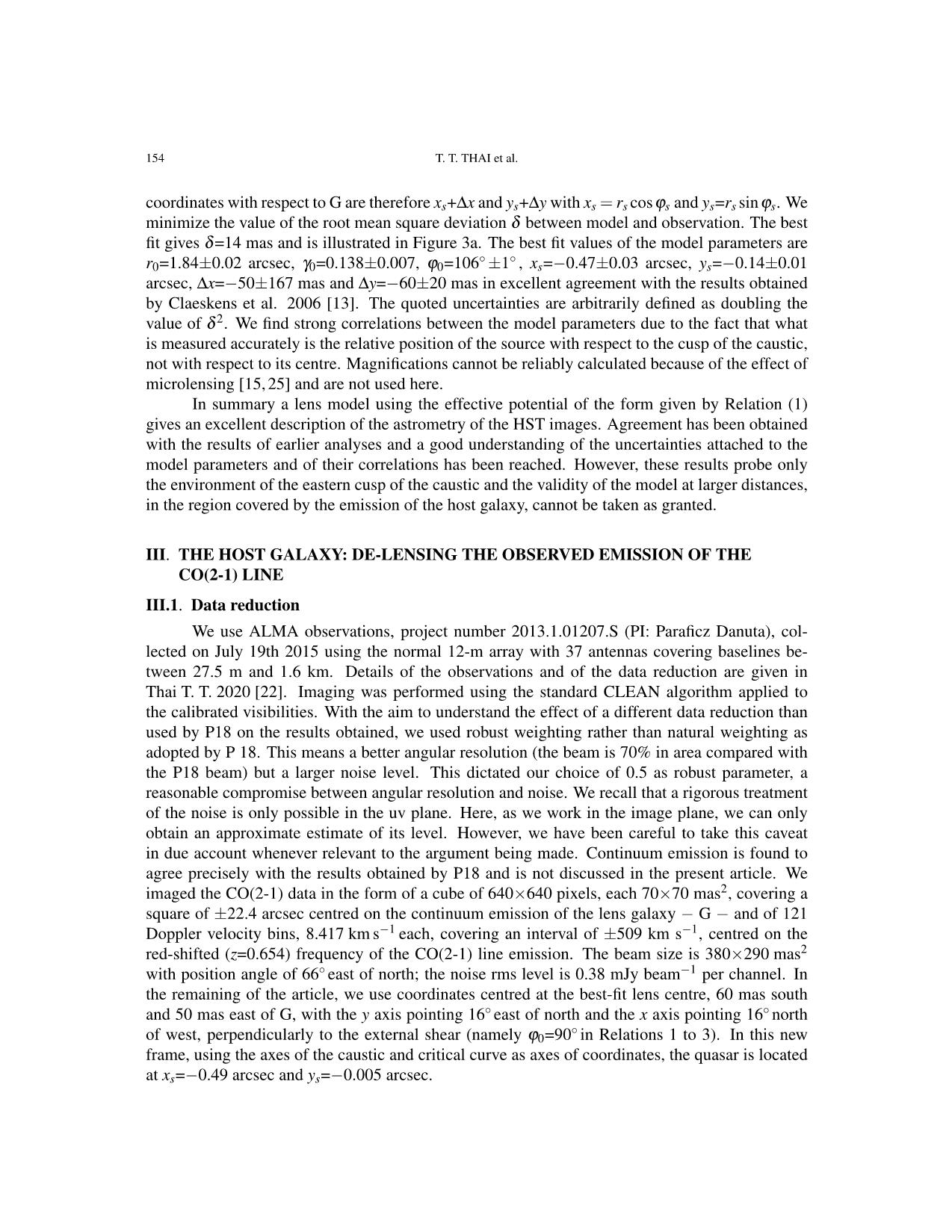
Trang 6
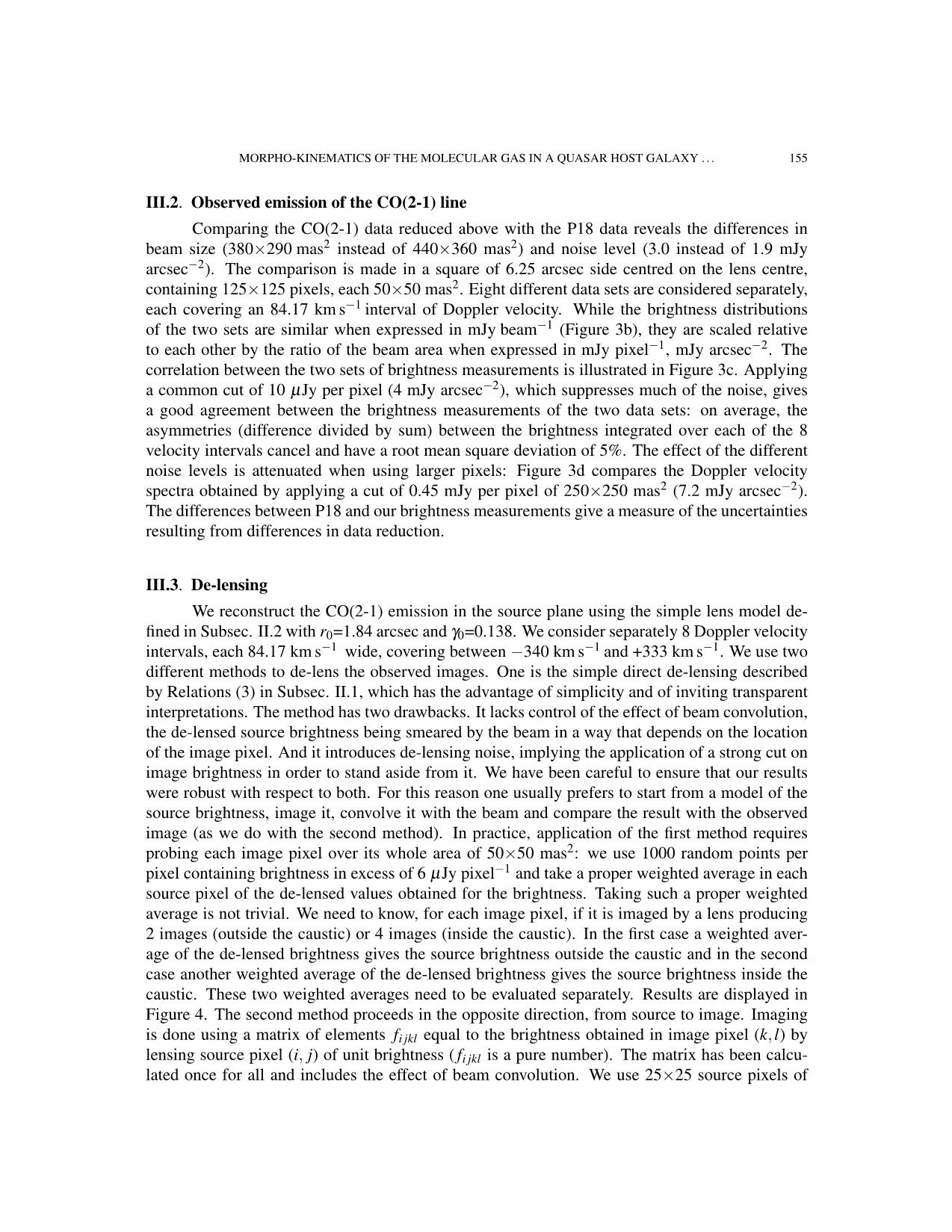
Trang 7
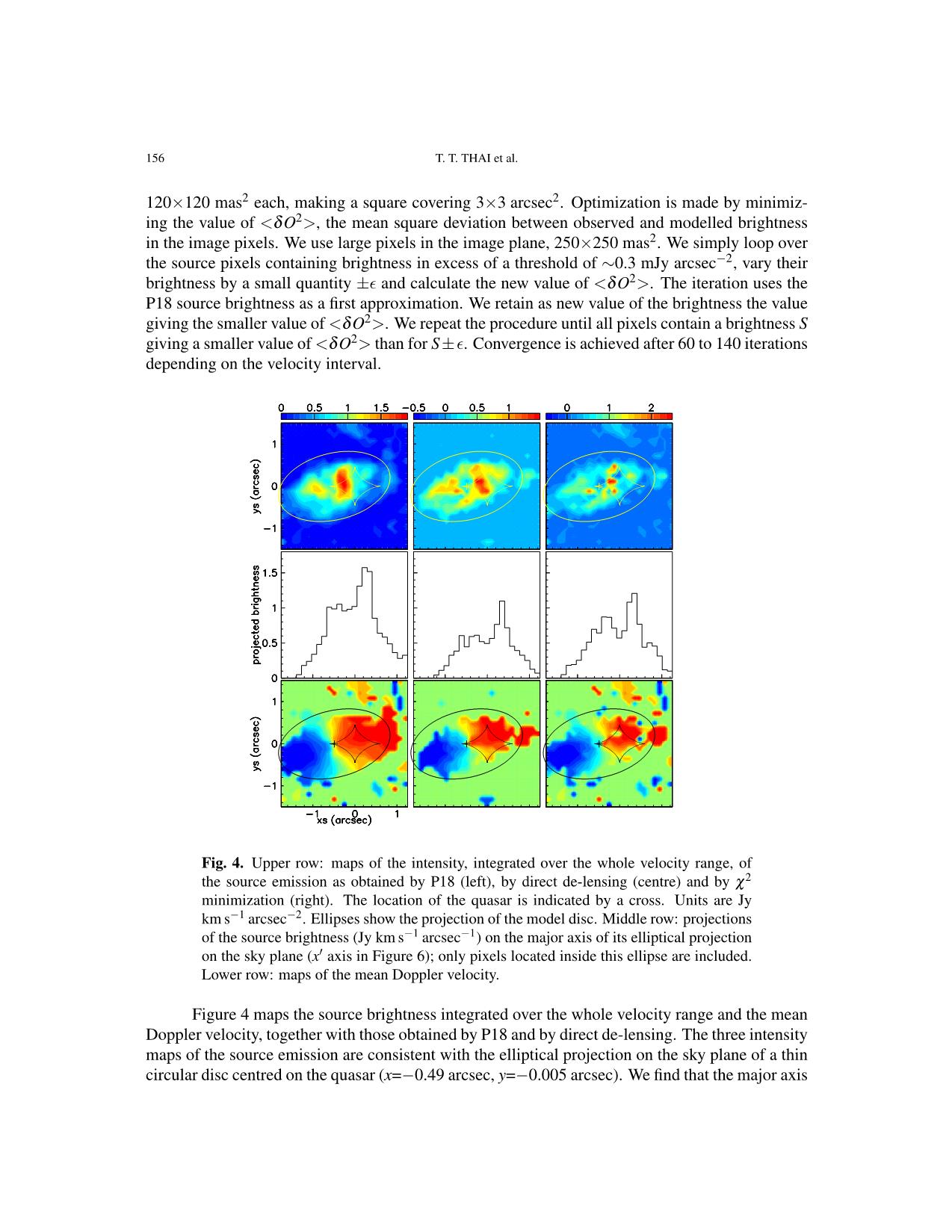
Trang 8
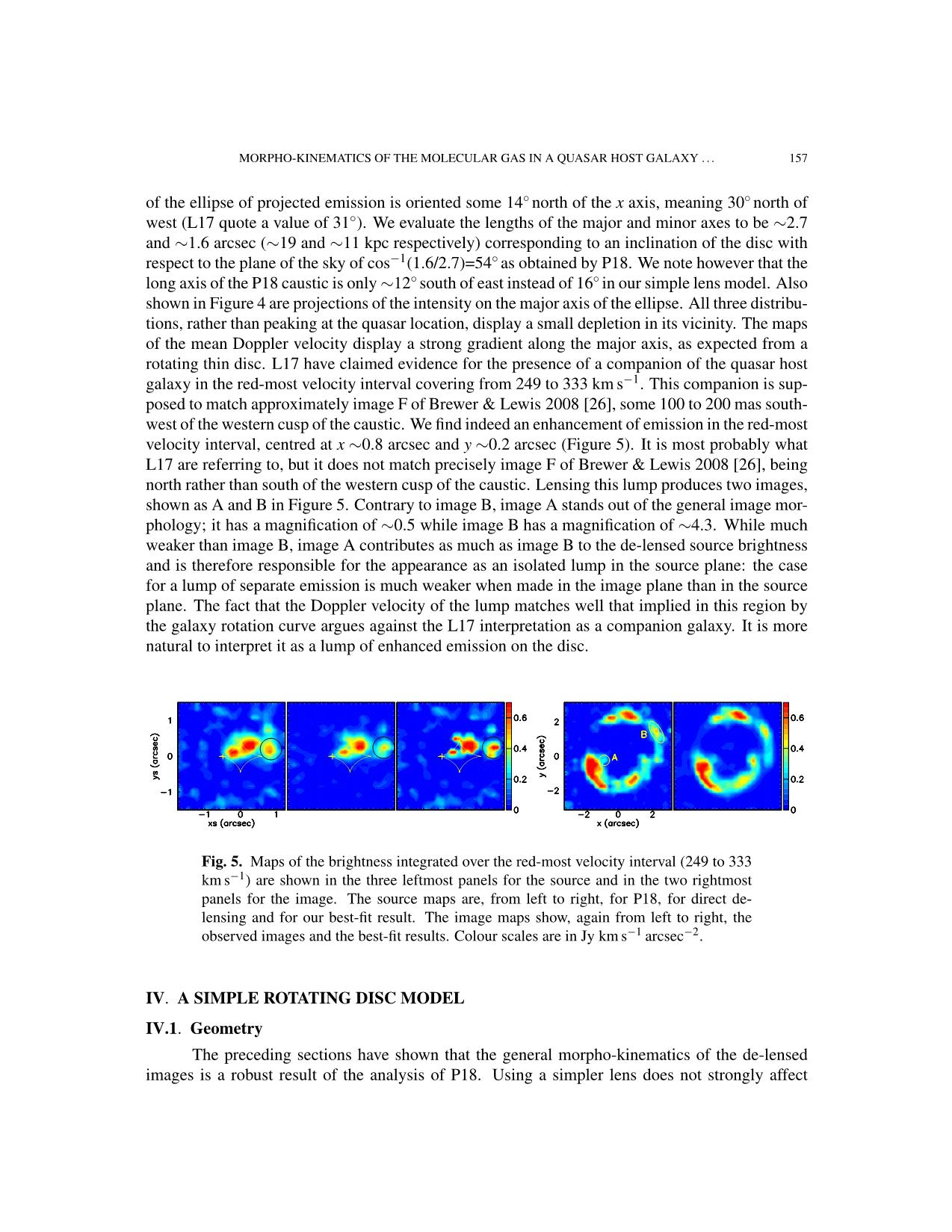
Trang 9
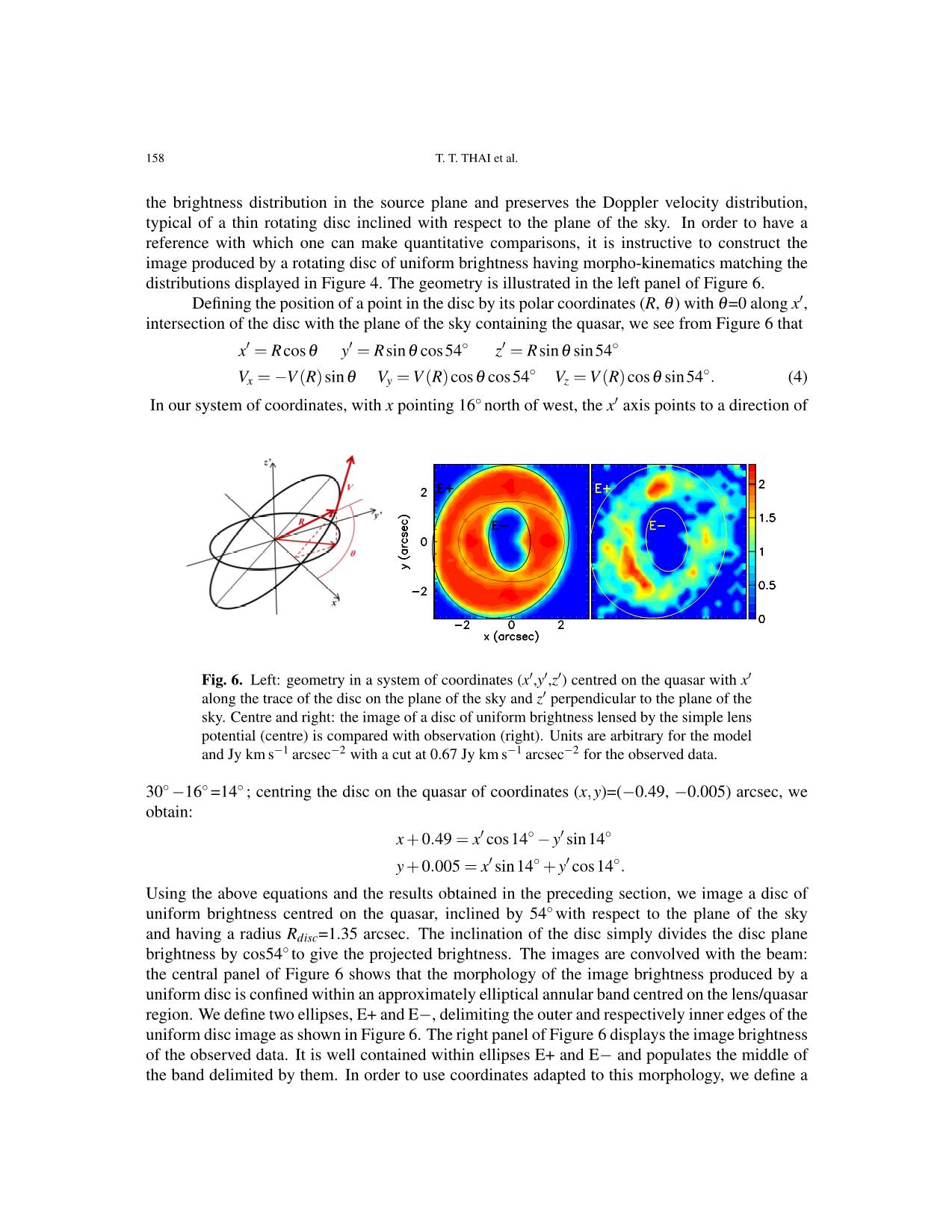
Trang 10
Tải về để xem bản đầy đủ
Tóm tắt nội dung tài liệu: Morpho-Kinematics of the molecular gas in a quasar host galaxy at redshift z = 0:654
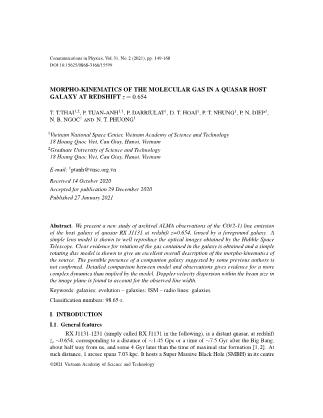
Communications in Physics, Vol.31, No. 2 (2021), pp. 149-168 DOI:10.15625/0868-3166/15599 MORPHO-KINEMATICS OF THE MOLECULAR GAS IN A QUASAR HOST GALAXY AT REDSHIFT z = 0.654 T. T.THAI1,2, P. TUAN-ANH1,†, P. DARRIULAT1, D. T. HOAI1, P. T. NHUNG1, P. N. DIEP1, N. B. NGOC1 AND N. T. PHUONG1 1Vietnam National Space Center, Vietnam Academy of Science and Technology 18 Hoang Quoc Viet, Cau Giay, Hanoi, Vietnam 2Graduate University of Science and Technology 18 Hoang Quoc Viet, Cau Giay, Hanoi, Vietnam E-mail: †ptanh@vnsc.org.vn Received 14 October 2020 Accepted for publication 29 December 2020 Published 27 January 2021 Abstract. We present a new study of archival ALMA observations of the CO(2-1) line emission of the host galaxy of quasar RX J1131 at redshift z=0.654, lensed by a foreground galaxy. A simple lens model is shown to well reproduce the optical images obtained by the Hubble Space Telescope. Clear evidence for rotation of the gas contained in the galaxy is obtained and a simple rotating disc model is shown to give an excellent overall description of the morpho-kinematics of the source. The possible presence of a companion galaxy suggested by some previous authors is not confirmed. Detailed comparison between model and observations gives evidence for a more complex dynamics than implied by the model. Doppler velocity dispersion within the beam size in the image plane is found to account for the observed line width. Keywords: galaxies: evolution – galaxies: ISM – radio lines: galaxies. Classification numbers: 98.65-r. I. INTRODUCTION I.1. General features RX J1131-1231 (simply called RX J1131 in the following), is a distant quasar, at redshift zs ∼0.654, corresponding to a distance of ∼1.45 Gpc or a time of ∼7.5 Gyr after the Big Bang, about half way from us, and some 4 Gyr later than the time of maximal star formation [1, 2]. At such distance, 1 arcsec spans 7.03 kpc. It hosts a Super Massive Black Hole (SMBH) in its centre ©2021 Vietnam Academy of Science and Technology 150 T. T. THAI et al. 8 with a mass of ∼2 10 M; it rotates extremely fast, reaching near half the light velocity [3]. The quasar and its host galaxy are gravitationally lensed by a galaxy in the foreground, at redshift zL ∼0.295. They are the object of numerous studies, in particular aiming at a better understanding of the cosmological parameters governing the expansion of the Universe ( [4, 5] and references therein). Microlensing caused by stars transiting across the line of sight to the quasar has been used to study the structure of the lens halo ( [6, 7] and references therein). Infrared observations obtained by Herschel [10] have measured the spectral energy distribu- Fig. 1. Left: dependence on the redshifts of the source (zS in abscissa) and of the lens (zL in ordinate) of the ratio between their respective angular diameter distances daS/daL [1,2]). The relative size of the lens with respect to the source is proportional to daS/daL. The stars show the locations of quasars RX J1131 (black, P18) and RX J0911 (red, [8], [9]). The sizes of the host galaxies are compared to the size of the lens caustic in the central and right panels respectively. tion (SED), and archival VLA observations (Program ID: AW741; PI: Wucknitz) analysed by Leung et al. 2017 [11], referred to as L17 in the following, have shown resolved continuum emis- sion from the jets and the core of the foreground elliptical galaxy as well as emission toward the background quasar. Thorough analyses of high angular resolution HST optical and NIR images [12–14] and of Keck Adaptive Optics images [15] have produced a detailed description of the lensing properties in the neighbourhood of the quasar. They reveal a typical long axis quad configuration [16,17], the quasar being located within the eastern cusp of the lens caustic. As emission from the lens galaxy is simultaneously detected, the parameters of the lensing potential can be accurately evaluated. However, they probe only the vicinity of the cusp of the caustic curve. As the emission of the quasar host galaxy covers the whole caustic curve and extends even farther out, one cannot take it as granted that the simple lens model obtained from the study of the quasar images reliably applies to the whole host galaxy. This is at variance with the gravitational lensing of quasar hosts that are farther away and cover only part of the caustic in addition to being intrinsically smaller. The central region of the caustic corresponds to images close to an Einstein ring configuration, which dominates the picture in the case of RX J1131. We illustrate this feature in Figure 1, which shows the location of RX J1131 in the plane zL vs zS, lens vs source redshifts, together with that of other multiple imaged systems [12], and compares it with the case of a typical farther away quasar host galaxy, RX J0911 [8, 9]. MORPHO-KINEMATICS OF TH ... The modelled spectra are obtained by de-lensing the images pro- duced by lensing the model disc source and convolved with the beam. Results are illustrated in the left panel of Figure 12. Qualitatively, the general trend is well reproduced by the model but significant differences are observed in the central segments: the data display larger Doppler ve- locities on the red side and lower Doppler velocities on the blue side than implied by the model. Moreover, in the central segment, the line width predicted by the model is much smaller than that observed in the data [22]. A natural interpretation of such an effect is disc warping causing an effective dependence on θ of the sine of the inclination angle in Relation (4). However, including warping in the model by writing Vz = V(R)cosθ sinϕ with ϕ depending simply on θ and R, gives only a modest improvement of the match between model and observations. This suggests that a more complex dynamics than described by the simple model is at stake. Beam convolution causes an effective smearing of the disc region contributing to each of the nine segments, making the radial distribution of the mean Doppler velocity measured in the central segments less steep and causing an increase of the velocity dispersion. This important result is a warning: measuring the velocity dispersion requires a careful evaluation of the contribution of rotation within the finite angular resolution of relevance. Making the angular acceptance smaller will decrease the direct contribution of rotation, independent from beam convolution, but will not decrease the contribution resulting from the smearing caused by the beam. This contribution is important: using the central segment as an example, the line width predicted by the model increases from 60 km s−1 to nearly 100 km s−1 when accounting for beam convolution, namely a beam contribution of over 70 km s−1 (both contributions add up in quadrature). 164 T. T. THAI et al. Fig. 11. Left panels display the brightness distribution in the source plane obtained by de-lensing depletion D (left) and excess E (centre-left) respectively. Right panels display 2 the dependence of χ on the factors FD and FE measuring the amplitudes of the depletion (centre-right) and excess (right) respectively when the other model parameters are fixed at their best-fit values. 0 Fig. 12. Dependence of on x (major axis of the projection of the disc on the plane of the sky) for the data (black) and the model (red) is shown in the left panel and the rotation curve in the right panel together with the L17 (blue curve) and P18 (red crosses) results. The differences between observed and predicted velocity dispersions may receive contribu- tions from the intrinsic line width (turbulence), which is absent from the model, or from different rotation contributions, as could be caused by disc warping. We recall that P18 claim that the obser- vation of a high dispersion in the vicinity of the quasar demonstrates that the region of enhanced emission studied in the preceding section is the seat of increased gas turbulence. However, the differences observed in the central segments of the band used to evaluate the rotation curve do not consist of a simple broadening of the line, as would be expected from turbulence, but reveal rather an excess of red-shifted emission. Maps of the intensity, mean Doppler velocity and Doppler velocity dispersion in the λ vs ω plane reveal important differences between model and observations [22]: predicted mean Doppler velocities tend to be too small in the 90◦<θ<180◦ quadrant and too large in the 270◦<θ<360◦ quadrant of the disc plane; velocity dispersions reach some 120 km s−1 for the model and some MORPHO-KINEMATICS OF THE MOLECULAR GAS IN A QUASAR HOST GALAXY ... 165 Fig. 13. Line profiles in the region |λ| <0.25 and 110◦<ω<250◦. Left: observations with cuts at 11.7 µJy (black) or 16 µJy (red); the lines show Gaussian fits with σ=85 km s−1 and 56 km s−1 respectively. Gaussian fits to the predictions of the disc model without (centre) and with (right) beam convolution give σ values of 18 km s−1 and 58 km s−1 respectively. 160 km s−1 for the observations. We failed to obtain a substantial improvement of the quality of the match between model and observations by including a simple description of disc warping in the model: this confirms the need for a more complex dynamics than implied by the simple disc model. From a number of comparisons between the line widths predicted by the model with and without beam convolution, the contribution is found to be in the range between 50 and 70 km s−1. An independent evaluation is obtained by selecting the central region of the λ vs ω plane (left column of Figure 10) defined as |λ| <0.25 and |ω − 180◦| <70◦. We segment it in 10×14 ◦ pixels of 0.05 in λ and 10 in ω. For each pixel (i, j) we evaluate the mean value i j of the Doppler velocity and show the distribution of Vz−i j in Figure 13. We obtain this way an evaluation of the line profile. The observed line widths (σ) are 85 and 56 km s−1 for cuts at 11.7 (1.5-σ) and 16 µJy (2-σ) per 50×50×8.417 mas2 km s−1 respectively. As the model includes no intrinsic line broadening, the line broadening shown by the model is entirely due to the contribution of rotation. Gaussian fits give σ values of 18 and 58 km s−1 without and with beam convolution respectively, implying a contribution of ∼50 km s−1 from the smearing effect of the beam size. The line width measured with the 16 µJy cut does not require any contribution of turbulence while that measured with an 11.7 µJy cut allows for a contribution reaching ∼60 km s−1: without a precise understanding of the contribution of noise, it is therefore difficult to obtain a reliable evaluation of the line broadening caused by turbulence. In summary, a simple rotating disc model gives a global picture that describes well the general trend of the observed kinematics. However, such a model fails to reproduce quantitatively the details of the Doppler velocity distribution: it reveals a more complex dynamics. Attempts to describe the mismatch as the result of a simple disc warping have failed. An important result of our analysis is that the smearing in the image plane caused by the beam size contributes between 50 and 70 km s−1 to the dispersion of the Doppler velocity. It combines in quadrature with the contribution of rotation within the angular acceptance being probed by the pixel size of relevance. Taken together, these effects make it difficult to evaluate reliably the contribution of turbulence to the line width. But they prevent claiming that such contribution is important: within experimental 166 T. T. THAI et al. uncertainties the line width can be accounted for by the dispersion of rotation velocities within the beam size. V. SUMMARY AND CONCLUSIONS The present study of the emission of the CO(2-1) molecular line by the host galaxy of quasar RX J1131 uses ALMA observations of unprecedented quality; they have been previously analysed in much detail by their proponents, [18], P18. We have shown that the HST images of the quasar and of the lens galaxy are very well reproduced by a simple lensing potential, sphere+external shear. We obtained parameters that are in agreement with the results of previous authors within errors. We discussed the uncertainties attached to the model parameters and their correlations and explained them by remarking that these results probe only the environment of the eastern cusp of the caustic. At this stage, the validity of the model at larger distances, in the region covered by the emission of the host galaxy, cannot be taken as granted. But we demonstrated its validity from our analysis of the ALMA observations of the CO(2-1) line emission. We reduced the raw data and produced cleaned images, at variance with P18 who perform their analysis in the uv plane. We obtained morpho-kinematics properties of the gas that are in good agreement with the results of P18. We used two different methods for de-lensing these images, which produce source brightness distributions in agreement with each other as well as with the P18 results. We have shown that the general morphology of the image is dictated by the properties of the lens and confined within a band bracketing the critical curve. This leaves in practice no freedom to conceive lensing potentials that would be significantly different from those that we and other authors have been using. It guarantees the robustness of the results obtained by P18 within observational uncertainties. We used polar coordinates better adapted to this geometry than Cartesians to reveal very clearly the evidence for rotation. We looked for possible deviations from the predictions of a simple rotating disc model, in particular for the possible presence of a companion as was predicted by L17 using Plateau de Bure observations of much less good angular resolution than the ALMA observations; but our analysis did not support such a presence. We paid special attention to the red- most velocity interval, which displays a particularly complex morphology. We found evidence for enhanced emission revealing a significant deviation from the prediction of the simple disc model. However the associated hot spot in the source plane overlaps the caustic, implying important uncertainties on its de-lensed properties. We discussed the rotation curve in some detail and argued that it probably rises faster than assumed by P18 in the vicinity of the quasar; we noted that the angular resolution is such that the dispersion of the rotation velocity within the beam size in the image plane causes an important effective broadening of the line width, which we evaluated in the range between 50 and 70 km s−1 . This is sufficient to account for the observed line width when applying a ∼2-σ cut on the data. However, uncertainties attached to the effect of noise prevent from giving a reliable evaluation of the contribution of turbulence. This is at variance with the conclusion of P18 who attribute the high Doppler velocity dispersion observed in the vicinity of the AGN to gas turbulence exclusively. P18 obtained from their analysis a number of results concerning the physical properties of the galaxy, which they compared with those of other similar galaxies. The nature of our study prevents us from adding much to their conclusions. We simply remark that their estimate of 11 the total dynamical mass enclosed within 5 kpc, (1.46±0.31)×10 M, may be affected by the steepness of the rotation curve: at fixed radius, the dynamical mass is proportional to the square of MORPHO-KINEMATICS OF THE MOLECULAR GAS IN A QUASAR HOST GALAXY ... 167 the rotation velocity. The analysis presented in the preceding sections implies therefore a possibly higher value of the gas mass, obtained by P18 and accordingly affect the value of the CO−H2 conversion factor. ACKNOWLEDGMENT We express our deep gratitude to Professors Frederic Courbin and Matus Rybak who kindly provided us with copies of data files summarizing the results of the P18 analysis. This paper uses archival ALMA data from project 2013.1.01207.S (PI: Paraficz Danuta). ALMA is a partnership of ESO (representing its member states), NSF (USA), NINS (Japan), NRC(Canada), NSC/ASIAA (Taiwan), and KASI (South Korea), in cooperation with Chile. The Joint ALMA Observatory is operated by ESO, AUI/NRAO and NAOJ. We are deeply indebted to the ALMA partnership, whose open access policy means invaluable support and encouragement for Vietnamese astro- physics. Financial support from the World Laboratory and VNSC is gratefully acknowledged. This research is funded by the Vietnam National Foundation for Science and Technology Devel- opment (NAFOSTED) under grant number 103.99-2019.368. REFERENCES [1] R. Adam, N. Aghanim, M. Ashdown, J. Aumont, C. Baccigalupi, M. Ballardini, A. Banday, R. Barreiro, N. Bar- tolo, S. Basak and R. Battye, A&A 596 (2016) A108. [2] P. A. Ade, N. Aghanim, M. Arnaud, M. Ashdown, J. Aumont, C. Baccigalupi, A. Banday, R. Barreiro, J. Bartlett, N. Bartolo et al., A&A 594 (2016) A13. [3] R. C. Reis, M. T. Reynolds, J. M. Miller and D. J. Walton, Nature 507 (2014) 207. [4] M. Tewes, F. Courbin, G. Meylan, C. Kochanek, E. Eulaers, N. Cantale, A. Mosquera, P. Magain, H. Van Winckel, D. Sluse and G. Cataldi, A&A 556 (2013) A22. [5] V. Bonvin, F. Courbin, S. H. Suyu, P. J. Marshall, C. E. Rusu, D. Sluse, M. Tewes, K. C. Wong, T. Collett, C. D. Fassnacht and T. Treu, Monthly Notices of the Royal Astronomical Society 465 (2017) 4914. [6] G. Chartas, C. S. Kochanek, X. Dai, D. Moore, A. M. Mosquera and J. A. Blackburne, ApJ 757 (2012) 137. [7] H. Sugai, A. Kawai, A. Shimono, T. Hattori, G. Kosugi, N. Kashikawa, K. T. Inoue and M. Chiba, ApJ 660 (2007) 1016. [8] P. Anh, F. Boone, D. Hoai, P. Nhung, A. Weiß, J.-P. Kneib, A. Beelen and P. Salome,´ A&A 552 (2013) L12. [9] A. P.T., PhD thesis,Vietnam Institute of Physics and Touluse University (2014) . [10] H. R. Stacey, J. P. McKean, N. C. Robertson, R. J. Ivison, K. G. Isaak, D. R. G. Schleicher, P. P. van der Werf, W. A. Baan, A. Berciano Alba, M. A. Garrett and A. F. Loenen, Mon. Notices Royal Astron. Soc. 476 (2018) 5075. [11] T. D. Leung, D. A. Riechers and R. Pavesi, ApJ 836 (2017) 180. [12] CASTLES, Summary of RXJ1131-1231 observations, RXJ1131.html, 2015, Mon Oct 26 19:54:39 EDT 2015. [13] J.-F. Claeskens, D. Sluse, P. Riaud and J. Surdej, A&A 451 (2006) 865. [14] S. Birrer, A. Amara and A. Refregier, J. Cosmol. Astropart. Phys. 2016 (2016) 020. [15] G. C.-F. Chen, S. H. Suyu, K. C. Wong, C. D. Fassnacht, T. Chiueh, A. Halkola, I. S. Hu, M. W. Auger, L. V. Koopmans, D. J. Lagattuta et al., Mon. Notices Royal Astron. Soc. 462 (2016) 3457. [16] R. Blandford, C. Kochanek, I. Kovner and R. Narayan, Science 245 (1989) 824. [17] P. Saha and L. L. R. Williams, AJ 125 (2003) 2769. [18] D. Paraficz, M. Rybak and J. P. McKean, A&A A34 (2018) 613. [19] M. Rybak, J. P. McKean, S. Vegetti, P. Andreani and S. D. M. White, Mon. Notices Royal Astron. Soc. 451 (2015) L40. [20] M. Rybak, S. Vegetti, J. P. McKean, P. Andreani and S. D. M. White, Mon. Notices Royal Astron. Soc. 453 (2015) L26. [21] S. Vegetti and L. V. E. Koopmans, Mon. Notices Royal Astron. Soc. 945 (2009) 392. 168 T. T. THAI et al. [22] T. T. Thai, Master thesis, Graduate University of Science and Technology (2020) . [23] P. T. Nhung, P. T. Anh, F. Boone, P. Darriulat, P. N. Diep, P. N. Dong, N. Van Hiep and N. T. Thao, Res. Astron. Astrophys. 13 (2013) 803. [24] S. H. Suyu, M. W. Auger and S. Hilbert, ApJ 70 (2013) 766. [25] D. Sluse, J.-F. Claeskens and B. Altieri, A&A 539 (2006) 449. [26] B. B. J. and G. F. Lewis, Mon. Notices Royal Astron. Soc. 39 (2008) 390.
File đính kèm:
morpho_kinematics_of_the_molecular_gas_in_a_quasar_host_gala.pdf