Effects of atomics growing orientation to mechanical properties of Cu/Ta bilayer using molecular dynamics simulation
Recently, metallic nanofilms are widely
studied because of their excellent mechanical
properties as follows: good toughness, high
strength, and great hardness, etc [1]. Besides,
metallic nanofilms are very easy in fabrication
and adjustment corresponding to different specific
conditions [2]. There are many factors that affect
the mechanical properties of metallic nanofilms,
however, atomics growing orientation directly
influence on the deformation mechanisms and
mechanical responses of metallic nanofilms under
testing processes. Therefore, in order to analyze
the changes in the structures of metallic nanofilms,
a careful and systematic study of the deformation
mechanisms and mechanical properties of nanofilms
in different atomics growing orientations conditions
is required.
Cu/Ta nanofilms is one of the most metallic
nanofilms commonly used in aerospace, electronic,
optical, and magnetic industries. Therefore, studying
the mechanical properties of Cu/Ta is a very urgent
requirement. However, it is very difficult to establish
a Cu/Ta nanofilm with different atomics growing
orientations by the experiment. With the strong
development of computer technology, molecular
dynamics (MD) simulation is a very accurate and
reasonable choice to investigate the properties of
materials at nanoscale [3-6].
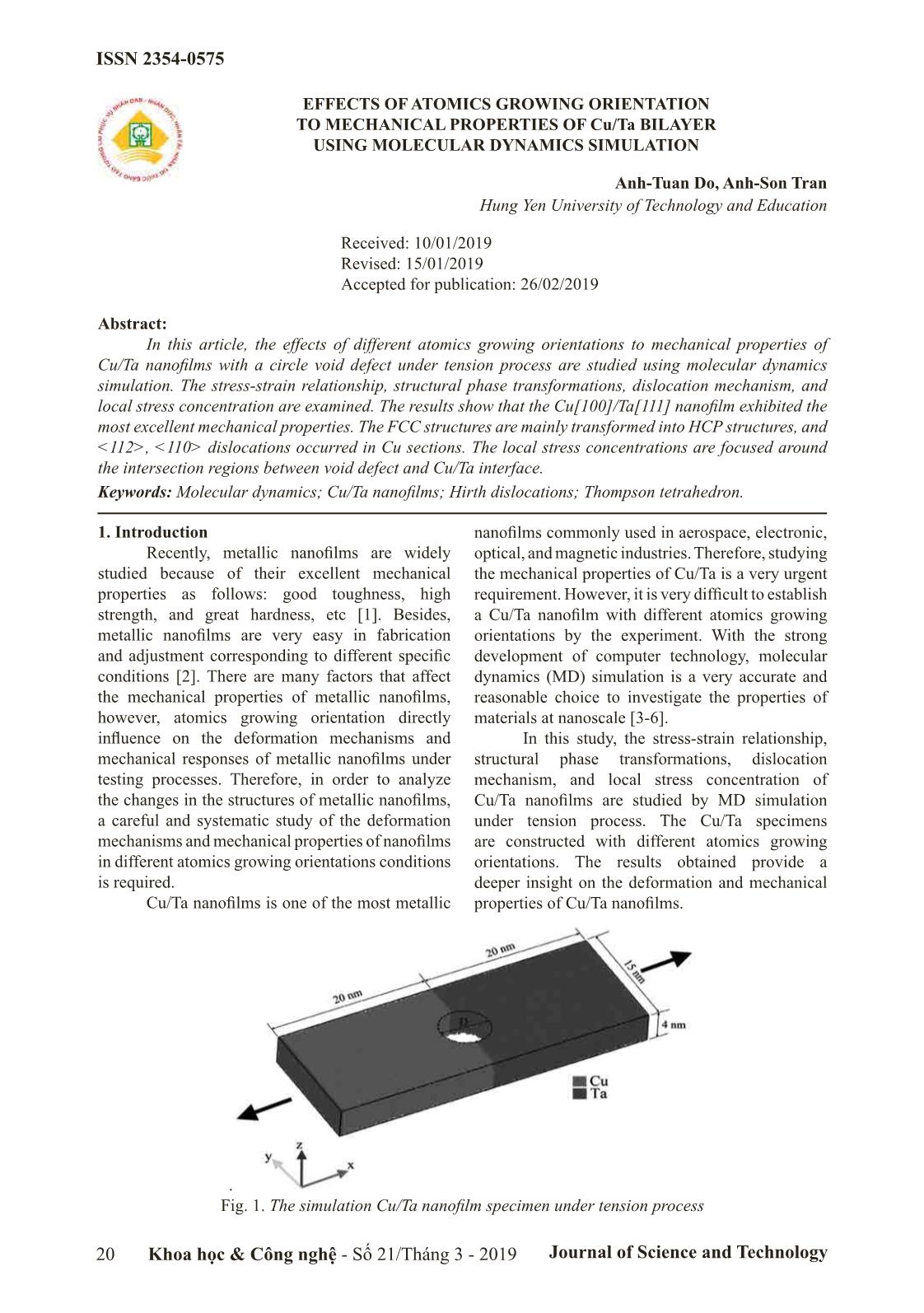
Trang 1
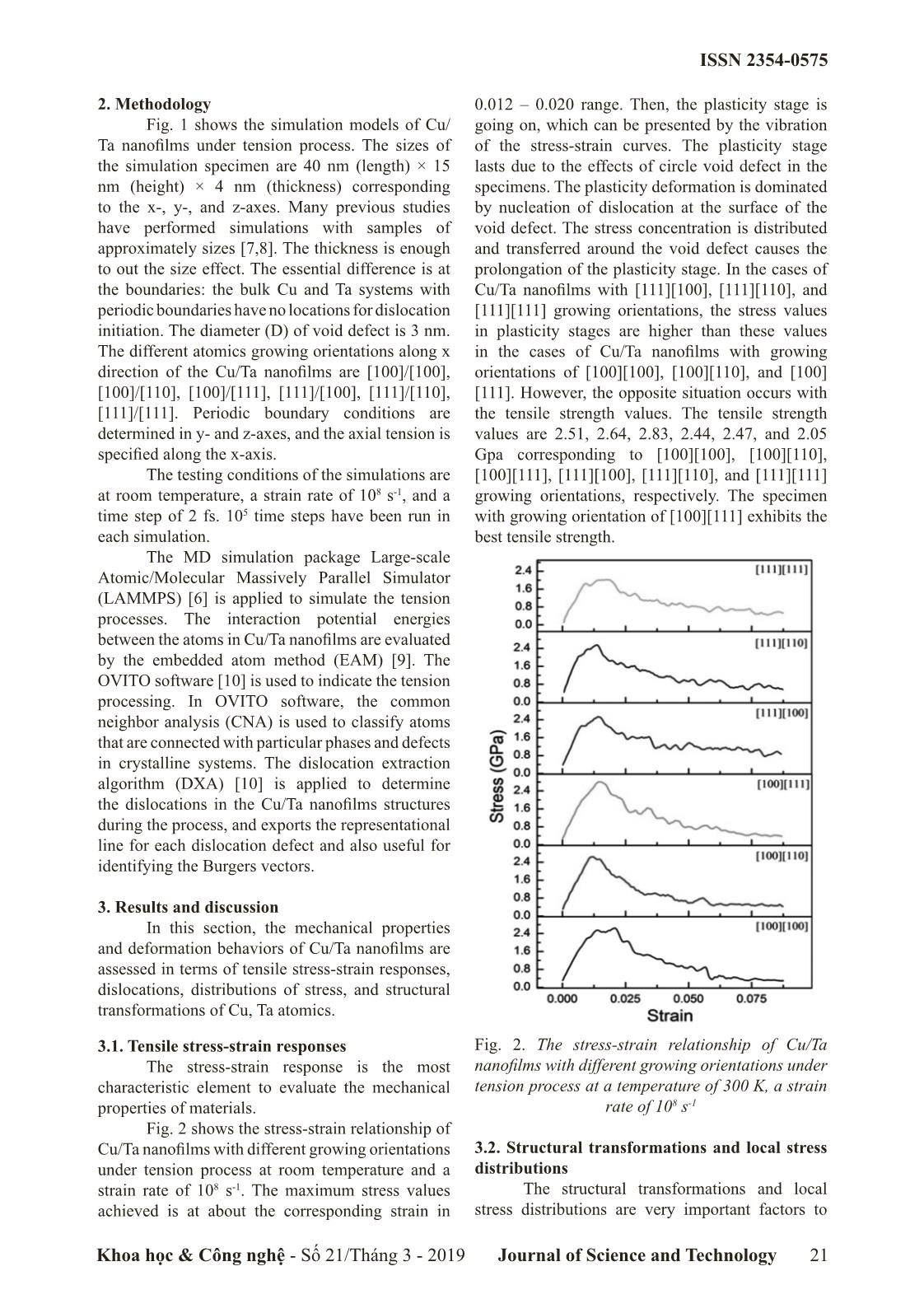
Trang 2
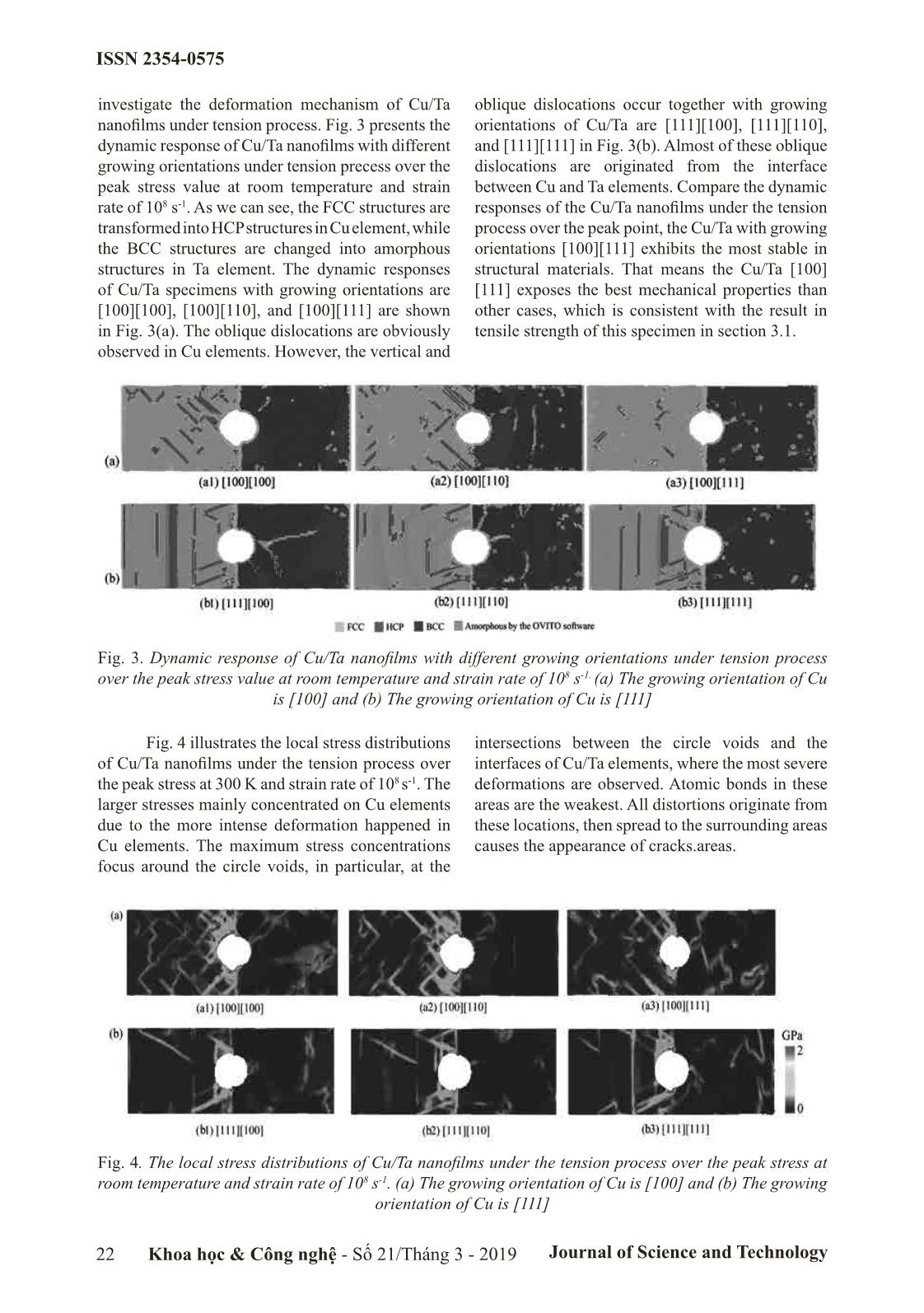
Trang 3
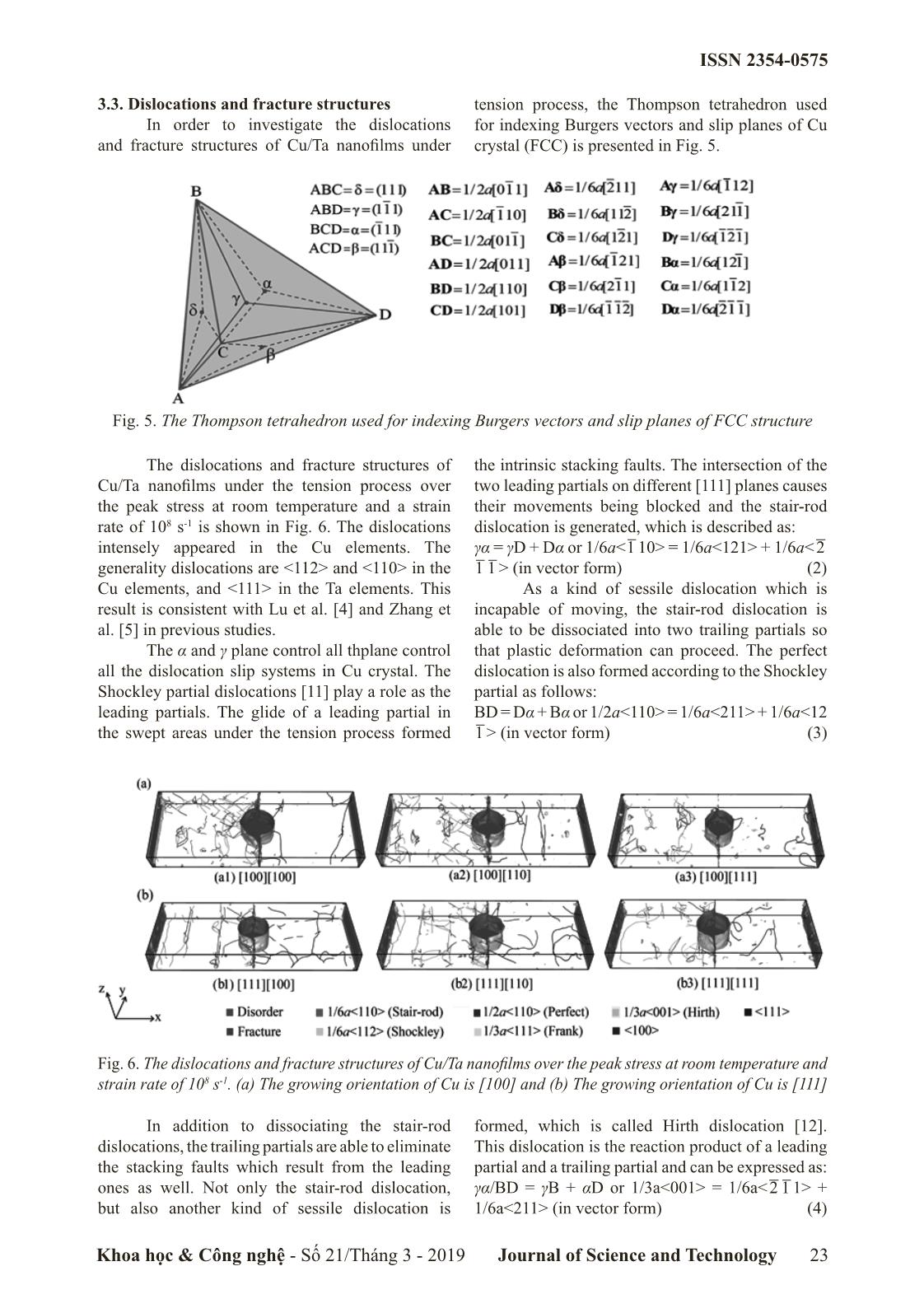
Trang 4
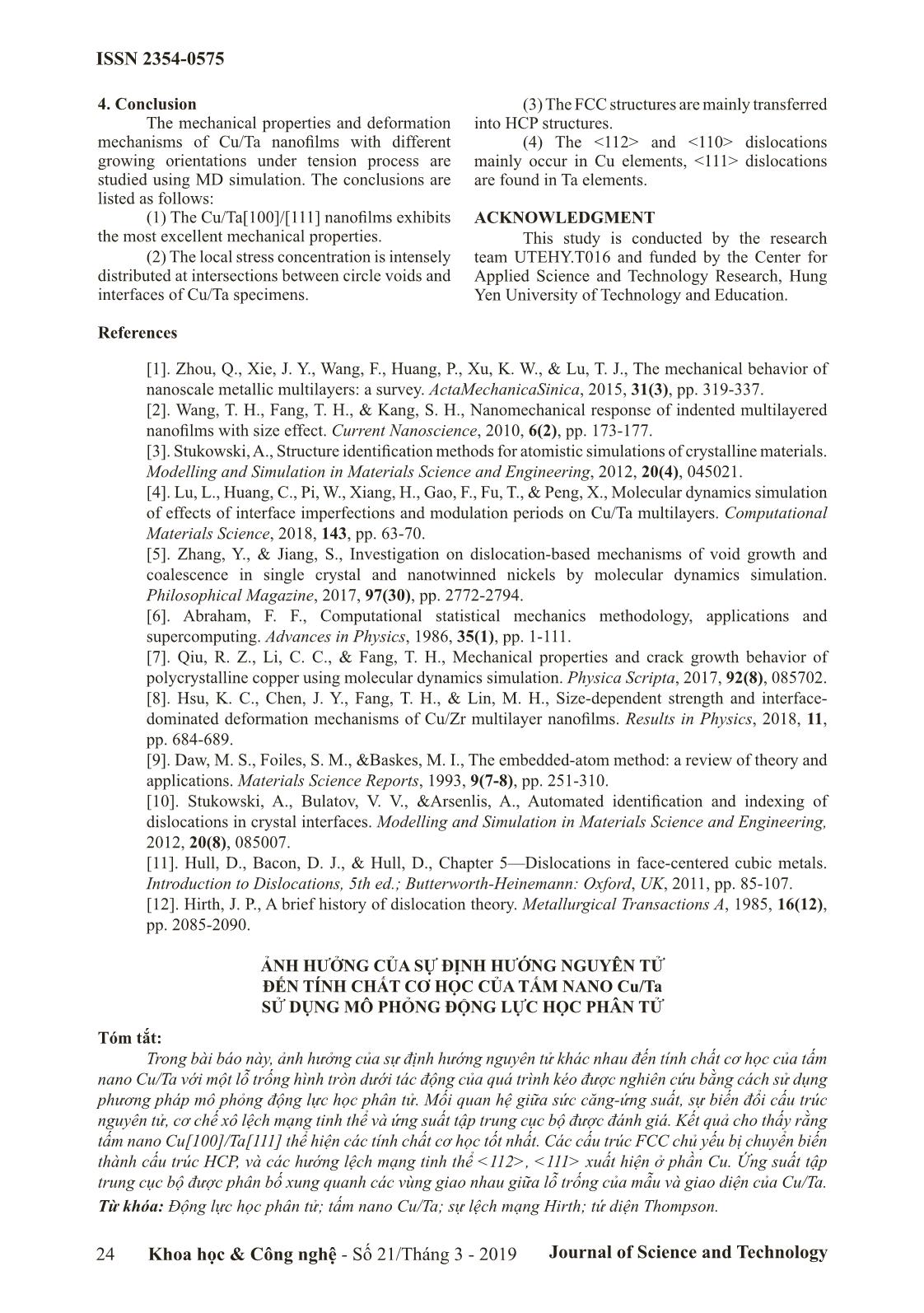
Trang 5
Tóm tắt nội dung tài liệu: Effects of atomics growing orientation to mechanical properties of Cu/Ta bilayer using molecular dynamics simulation
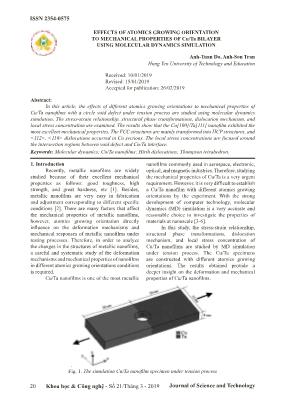
ISSN 2354-0575 EFFECTS OF ATOMICS GROWING ORIENTATION TO MECHANICAL PROPERTIES OF Cu/Ta BILAYER USING MOLECULAR DYNAMICS SIMULATION Anh-Tuan Do, Anh-Son Tran Hung Yen University of Technology and Education Received: 10/01/2019 Revised: 15/01/2019 Accepted for publication: 26/02/2019 Abstract: In this article, the effects of different atomics growing orientations to mechanical properties of Cu/Ta nanofilms with a circle void defect under tension process are studied using molecular dynamics simulation. The stress-strain relationship, structural phase transformations, dislocation mechanism, and local stress concentration are examined. The results show that the Cu[100]/Ta[111] nanofilm exhibited the most excellent mechanical properties. The FCC structures are mainly transformed into HCP structures, and , dislocations occurred in Cu sections. The local stress concentrations are focused around the intersection regions between void defect and Cu/Ta interface. Keywords: Molecular dynamics; Cu/Ta nanofilms; Hirth dislocations; Thompson tetrahedron. 1. Introduction nanofilms commonly used in aerospace, electronic, Recently, metallic nanofilms are widely optical, and magnetic industries. Therefore, studying studied because of their excellent mechanical the mechanical properties of Cu/Ta is a very urgent properties as follows: good toughness, high requirement. However, it is very difficult to establish strength, and great hardness, etc [1]. Besides, a Cu/Ta nanofilm with different atomics growing metallic nanofilms are very easy in fabrication orientations by the experiment. With the strong and adjustment corresponding to different specific development of computer technology, molecular conditions [2]. There are many factors that affect dynamics (MD) simulation is a very accurate and the mechanical properties of metallic nanofilms, reasonable choice to investigate the properties of however, atomics growing orientation directly materials at nanoscale [3-6]. influence on the deformation mechanisms and In this study, the stress-strain relationship, mechanical responses of metallic nanofilms under structural phase transformations, dislocation testing processes. Therefore, in order to analyze mechanism, and local stress concentration of the changes in the structures of metallic nanofilms, Cu/Ta nanofilms are studied by MD simulation a careful and systematic study of the deformation under tension process. The Cu/Ta specimens mechanisms and mechanical properties of nanofilms are constructed with different atomics growing in different atomics growing orientations conditions orientations. The results obtained provide a is required. deeper insight on the deformation and mechanical Cu/Ta nanofilms is one of the most metallic properties of Cu/Ta nanofilms. . Fig. 1. The simulation Cu/Ta nanofilm specimen under tension process 20 Khoa học & Công nghệ - Số 21/Tháng 3 - 2019 Journal of Science and Technology ISSN 2354-0575 2. Methodology 0.012 – 0.020 range. Then, the plasticity stage is Fig. 1 shows the simulation models of Cu/ going on, which can be presented by the vibration Ta nanofilms under tension process. The sizes of of the stress-strain curves. The plasticity stage the simulation specimen are 40 nm (length) × 15 lasts due to the effects of circle void defect in the nm (height) × 4 nm (thickness) corresponding specimens. The plasticity deformation is dominated to the x-, y-, and z-axes. Many previous studies by nucleation of dislocation at the surface of the have performed simulations with samples of void defect. The stress concentration is distributed approximately sizes [7,8]. The thickness is enough and transferred around the void defect causes the to out the size effect. The essential difference is at prolongation of the plasticity stage. In the cases of the boundaries: the bulk Cu and Ta systems with Cu/Ta nanofilms with [111][100], [111][110], and periodic boundaries have no locations for dislocation [111][111] growing orientations, the stress values initiation. The diameter (D) of void defect is 3 nm. in plasticity stages are higher than these values The different atomics growing orientations along x in the cases of Cu/Ta nanofilms with growing direction of the Cu/Ta nanofilms are [100]/[100], orientations of [100][100], [100][110], and [100] [100]/[110], [100]/[111], [111]/[100], [111]/[110], [111]. However, the opposite situation occurs with [111]/[111]. Periodic boundary conditions are the tensile strength values. The tensile strength determined in y- and z-axes, and the axial tension is values are 2.51, 2.64, 2.83, 2.44, 2.47, and 2.05 specified along the x-axis. Gpa corresponding to [100][100], [100][110], The testing conditions of the simulations are [100][111], [111][100], [111][110], and [111][111] at room temperature, a strain rate of 108 s-1, and a growing orientations, respectively. The specimen time step of 2 fs. 105 time steps have been run in with growing orientation of [100][111] exhibits the each simulation. best tensile strength. The MD simulation package Large-scale Atomic/Molecular Massively Parallel Simulator (LAMMPS) [6] is applied to simulate the tension processes. The interaction potential energies between the atoms in Cu/Ta nanofilms are evaluated by the embedded atom method (EAM) [9]. The OVITO software [10] is used to indicate the tension processing. In OVITO software, the common neighbor analysis (CNA) is used to classify atoms that are connected with particular phases and defects in crystalline systems. The dislocation extraction algorithm (DXA) [10] is applied to determine the dislocations in the Cu/Ta nanofilms structures during the process, and exports the representational line for each dislocation defect and also useful for identifying the Burgers vectors. 3. Results and discussion In this section, the mechanical properties and deformation behaviors of Cu/Ta nanofilms are assessed in terms of tensile stress-strain responses, dislocations, distributions of stress, and structural transformations of Cu, Ta atomics. 3.1. Tensile stress-strain responses Fig. 2. The stress-strain relationship of Cu/Ta The stress-strain response is the most nanofilms with different growing orientations under characteristic element to evaluate the mechanical tension process at a temperature of 300 K, a strain properties of materials. rate of 108 s-1 Fig. 2 shows the stress-strain relationship of Cu/Ta nanofilms with different growing orientations 3.2. Structural transformations and local stress under tension process at room temperature and a distributions strain rate of 108 s-1. The maximum stress values The structural transformations and local achieved is at about the corresponding strain in stress distributions are very important factors to Khoa học & Công nghệ - Số 21/Tháng 3 - 2019 Journal of Science and Technology 21 ISSN 2354-0575 investigate the deformation mechanism of Cu/Ta oblique dislocations occur together with growing nanofilms under tension process. Fig. 3 presents the orientations of Cu/Ta are [111][100], [111][110], dynamic response of Cu/Ta nanofilms with different and [111][111] in Fig. 3(b). Almost of these oblique growing orientations under tension precess over the dislocations are originated from the interface peak stress value at room temperature and strain between Cu and Ta elements. Compare the dynamic rate of 108 s-1. As we can see, the FCC structures are responses of the Cu/Ta nanofilms under the tension transformed into HCP structures in Cu element, while process over the peak point, the Cu/Ta with growing the BCC structures are changed into amorphous orientations [100][111] exhibits the most stable in structures in Ta element. The dynamic responses structural materials. That means the Cu/Ta [100] of Cu/Ta specimens with growing orientations are [111] exposes the best mechanical properties than [100][100], [100][110], and [100][111] are shown other cases, which is consistent with the result in in Fig. 3(a). The oblique dislocations are obviously tensile strength of this specimen in section 3.1. observed in Cu elements. However, the vertical and Fig. 3. Dynamic response of Cu/Ta nanofilms with different growing orientations under tension process over the peak stress value at room temperature and strain rate of 108 s-1. (a) The growing orientation of Cu is [100] and (b) The growing orientation of Cu is [111] Fig. 4 illustrates the local stress distributions intersections between the circle voids and the of Cu/Ta nanofilms under the tension process over interfaces of Cu/Ta elements, where the most severe the peak stress at 300 K and strain rate of 108 s-1. The deformations are observed. Atomic bonds in these larger stresses mainly concentrated on Cu elements areas are the weakest. All distortions originate from due to the more intense deformation happened in these locations, then spread to the surrounding areas Cu elements. The maximum stress concentrations causes the appearance of cracks.areas. focus around the circle voids, in particular, at the Fig. 4. The local stress distributions of Cu/Ta nanofilms under the tension process over the peak stress at room temperature and strain rate of 108 s-1. (a) The growing orientation of Cu is [100] and (b) The growing orientation of Cu is [111] 22 Khoa học & Công nghệ - Số 21/Tháng 3 - 2019 Journal of Science and Technology ISSN 2354-0575 3.3. Dislocations and fracture structures tension process, the Thompson tetrahedron used In order to investigate the dislocations for indexing Burgers vectors and slip planes of Cu and fracture structures of Cu/Ta nanofilms under crystal (FCC) is presented in Fig. 5. Fig. 5. The Thompson tetrahedron used for indexing Burgers vectors and slip planes of FCC structure The dislocations and fracture structures of the intrinsic stacking faults. The intersection of the Cu/Ta nanofilms under the tension process over two leading partials on different [111] planes causes the peak stress at room temperature and a strain their movements being blocked and the stair-rod rate of 108 s-1 is shown in Fig. 6. The dislocations dislocation is generated, which is described as: intensely appeared in the Cu elements. The γα = γD + Dα or 1/6a = 1/6a + 1/6a<2 generality dislocations are and in the 1 1 > (in vector form) (2) Cu elements, and in the Ta elements. This As a kind of sessile dislocation which is result is consistent with Lu et al. [4] and Zhang et incapable of moving, the stair-rod dislocation is al. [5] in previous studies. able to be dissociated into two trailing partials so The α and γ plane control all thplane control that plastic deformation can proceed. The perfect all the dislocation slip systems in Cu crystal. The dislocation is also formed according to the Shockley Shockley partial dislocations [11] play a role as the partial as follows: leading partials. The glide of a leading partial in BD = Dα + Bα or 1/2a = 1/6a + 1/6a<12 the swept areas under the tension process formed 1 > (in vector form) (3) Fig. 6. The dislocations and fracture structures of Cu/Ta nanofilms over the peak stress at room temperature and strain rate of 108 s-1. (a) The growing orientation of Cu is [100] and (b) The growing orientation of Cu is [111] In addition to dissociating the stair-rod formed, which is called Hirth dislocation [12]. dislocations, the trailing partials are able to eliminate This dislocation is the reaction product of a leading the stacking faults which result from the leading partial and a trailing partial and can be expressed as: ones as well. Not only the stair-rod dislocation, γα/BD = γB + αD or 1/3a = 1/6a + but also another kind of sessile dislocation is 1/6a (in vector form) (4) Khoa học & Công nghệ - Số 21/Tháng 3 - 2019 Journal of Science and Technology 23 ISSN 2354-0575 4. Conclusion (3) The FCC structures are mainly transferred The mechanical properties and deformation into HCP structures. mechanisms of Cu/Ta nanofilms with different (4) The and dislocations growing orientations under tension process are mainly occur in Cu elements, dislocations studied using MD simulation. The conclusions are are found in Ta elements. listed as follows: (1) The Cu/Ta[100]/[111] nanofilms exhibits ACKNOWLEDGMENT the most excellent mechanical properties. This study is conducted by the research (2) The local stress concentration is intensely team UTEHY.T016 and funded by the Center for distributed at intersections between circle voids and Applied Science and Technology Research, Hung interfaces of Cu/Ta specimens. Yen University of Technology and Education. References [1]. Zhou, Q., Xie, J. Y., Wang, F., Huang, P., Xu, K. W., & Lu, T. J., The mechanical behavior of nanoscale metallic multilayers: a survey. ActaMechanicaSinica, 2015, 31(3), pp. 319-337. [2]. Wang, T. H., Fang, T. H., & Kang, S. H., Nanomechanical response of indented multilayered nanofilms with size effect. Current Nanoscience, 2010, 6(2), pp. 173-177. [3]. Stukowski, A., Structure identification methods for atomistic simulations of crystalline materials. Modelling and Simulation in Materials Science and Engineering, 2012, 20(4), 045021. [4]. Lu, L., Huang, C., Pi, W., Xiang, H., Gao, F., Fu, T., & Peng, X., Molecular dynamics simulation of effects of interface imperfections and modulation periods on Cu/Ta multilayers. Computational Materials Science, 2018, 143, pp. 63-70. [5]. Zhang, Y., & Jiang, S., Investigation on dislocation-based mechanisms of void growth and coalescence in single crystal and nanotwinned nickels by molecular dynamics simulation. Philosophical Magazine, 2017, 97(30), pp. 2772-2794. [6]. Abraham, F. F., Computational statistical mechanics methodology, applications and supercomputing. Advances in Physics, 1986, 35(1), pp. 1-111. [7]. Qiu, R. Z., Li, C. C., & Fang, T. H., Mechanical properties and crack growth behavior of polycrystalline copper using molecular dynamics simulation. Physica Scripta, 2017, 92(8), 085702. [8]. Hsu, K. C., Chen, J. Y., Fang, T. H., & Lin, M. H., Size-dependent strength and interface- dominated deformation mechanisms of Cu/Zr multilayer nanofilms. Results in Physics, 2018, 11, pp. 684-689. [9]. Daw, M. S., Foiles, S. M., &Baskes, M. I., The embedded-atom method: a review of theory and applications. Materials Science Reports, 1993, 9(7-8), pp. 251-310. [10]. Stukowski, A., Bulatov, V. V., &Arsenlis, A., Automated identification and indexing of dislocations in crystal interfaces. Modelling and Simulation in Materials Science and Engineering, 2012, 20(8), 085007. [11]. Hull, D., Bacon, D. J., & Hull, D., Chapter 5—Dislocations in face-centered cubic metals. Introduction to Dislocations, 5th ed.; Butterworth-Heinemann: Oxford, UK, 2011, pp. 85-107. [12]. Hirth, J. P., A brief history of dislocation theory. Metallurgical Transactions A, 1985, 16(12), pp. 2085-2090. ẢNH HƯỞNG CỦA SỰ ĐỊNH HƯỚNG NGUYÊN TỬ ĐẾN TÍNH CHẤT CƠ HỌC CỦA TẤM NANO Cu/Ta SỬ DỤNG MÔ PHỎNG ĐỘNG LỰC HỌC PHÂN TỬ Tóm tắt: Trong bài báo này, ảnh hưởng của sự định hướng nguyên tử khác nhau đến tính chất cơ học của tấm nano Cu/Ta với một lỗ trống hình tròn dưới tác động của quá trình kéo được nghiên cứu bằng cách sử dụng phương pháp mô phỏng động lực học phân tử. Mối quan hệ giữa sức căng-ứng suất, sự biến đổi cấu trúc nguyên tử, cơ chế xô lệch mạng tinh thể và ứng suất tập trung cục bộ được đánh giá. Kết quả cho thấy rằng tấm nano Cu[100]/Ta[111] thể hiện các tính chất cơ học tốt nhất. Các cấu trúc FCC chủ yếu bị chuyển biến thành cấu trúc HCP, và các hướng lệch mạng tinh thể , xuất hiện ở phần Cu. Ứng suất tập trung cục bộ được phân bố xung quanh các vùng giao nhau giữa lỗ trống của mẫu và giao diện của Cu/Ta. Từ khóa: Động lực học phân tử; tấm nano Cu/Ta; sự lệch mạng Hirth; tứ diện Thompson. 24 Khoa học & Công nghệ - Số 21/Tháng 3 - 2019 Journal of Science and Technology
File đính kèm:
effects_of_atomics_growing_orientation_to_mechanical_propert.pdf